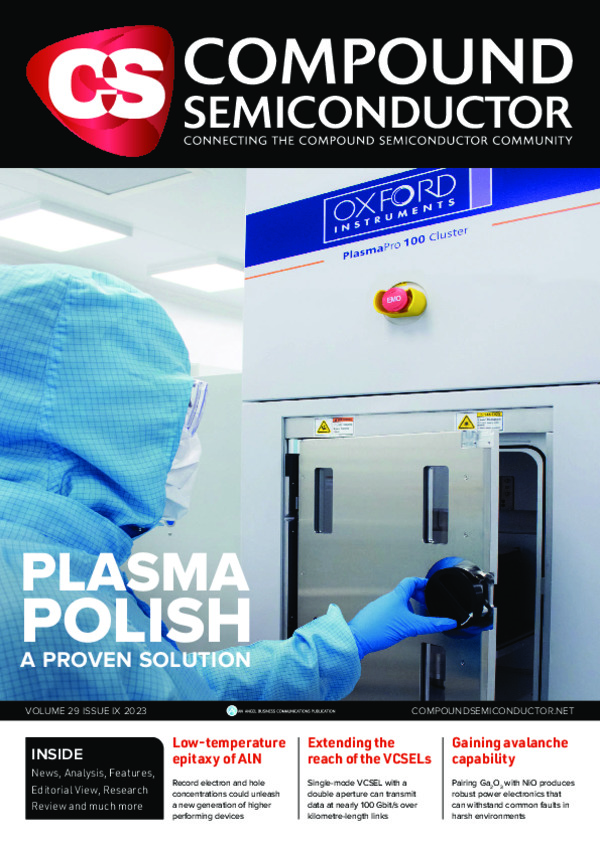
Extending the reach of high-speed VCSELs
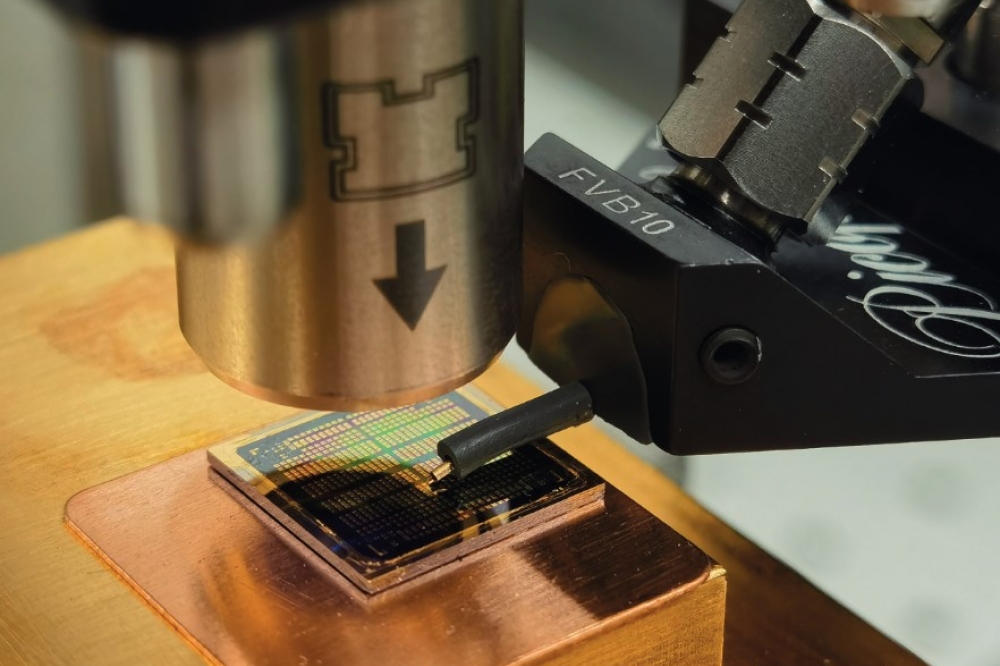
Single-mode oxide-VCSELs incorporating a double aperture can transmit data at nearly 100 Gbit/s over 1 km links.
BY
HAONAN WU, DUFEI WU AND MILTON FENG FROM UNIVERSITY OF ILLINOIS AT
URBANA-CHAMPAIGN AND XIN YU FROM FOXCONN INTERCONNECT TECHNOLOGY
Today the VCSEL is probably best known for its widespread use as a proximity sensor in smartphones. But its first high-volume market is datacoms, a sector that continues to provide substantial sales to many makers of this class of laser.
For more than twenty years ultra-low-power, oxide-confined VCSELs, which use an oxide aperture to confine the emission, have provided a cost-effective and scalable solution to short-reach optical interconnects in data centers. Supporting links of no more than 100 m or so, these devices combine low-power consumption with a compact form factor and high-speed data transfer. It’s a set of attributes that assist in the efficient operation of data centre networks.
Unfortunately, conventional multi-mode oxide VCSEL cannot be deployed to transmit data over longer lengths of fibre. Due to differential modal delay and chromatic dispersion in multi-mode fibre, the multi-mode nature of the emission makes this device unsuitable for optical data transmission over distances of several hundred metres or more. Transmission over such distances is needed, for example, to connect one hub to another.
Figure 1. Cross-section view of the integrated-mode-select-filter (IMSF)
mesa structure and bottom oxide aperture of the fabricated single-mode
VCSEL. The single transverse-mode operation is achieved with an
optimised top and bottom aperture ratio and IMSF height. The scanning
electron microscopy image is the fabricated device sliced by the focused
ion beam.
One option for extending transmission is to turn to a single-mode VCSEL. Using this approach, a team from Chalmers University of Technology, Sweden, reported 20 Gbit/s non-return-to-zero data transmission for link lengths of up to 2 km. However, that group’s approach to realising single-mode emission involved scaling the oxide-aperture. This has downsides, including a compromised reliability and a thermally limited laser operating current at a high modulation bandwidth.
What’s clear is that there’s a need to develop high-performance, single-mode VCSELs, as they are a vital ingredient to trimming the cost of emerging technologies, such as 5G networks, where high-speed and low-latency data transmission is critical for wireless backhaul and fronthaul connections. Success on this front would also create sources with a circular beam profile that are compatible with standard single-mode optical fibre. Such interoperability simplifies the integration of single-mode VCSELs into existing long-distance optical communication networks, making them a practical, cost-efficient choice for network upgrades and expansions.
A novel design
Breaking new ground on this front is a form of VCSEL with an innovative integrated-mode-select-filter, a design pioneered by our collaboration between the University of Illinois at Urbana-Champaign and sponsored by Foxconn Interconnect Technology.
In terms of fabrication, a key difference between our design and that of a conventional VCSEL is that ours requires a two-step oxidation process to form the top and bottom oxide apertures (see Figure 1, which provides a cross-sectional view of the device). Aside from that, our design is similar to that of a conventional oxide-VCSEL – it features a half-wavelength cavity, a p-doped distributed Bragg reflector mesa, an electrical current/optical confinement oxide layer, and contact metals.
To create the top oxide aperture, we oxidise multiple p-type pairs in the distributed Bragg reflector. This is followed by the formation of the integrated-mode-select-filter mesa, which is around 6 µm in diameter, and defined by a calibrated, chlorine-based dry etch using reactive ions and an inductively coupled plasma. By fine-tuning the composition of the dry etching gas, adjusting the plasma pressure, and optimising the ratio of reactive ion etch to the power of the inductively coupled plasma, we realise precise control over the sidewall of the mesa. This enables us to produce smooth sidewalls that maintain a 90-degree orientation relative to the p-type distributed Bragg reflector mesa. Note that due to difficulty in controlling the oxidation rate of this mesa, it is essential to have precise dry-etching process control, as this is key to defining the top oxide aperture size. Another key to a high yield across the entire sample is accurate control of the top and bottom oxide aperture ratio.
Figure 2. Measured optical spectrum of the single-mode VCSEL. The side-mode suppression ratio exceeds 34 dB.
Thanks to the unique mesa structure in our novel VCSEL, this design favours the lasing condition of the fundamental mode (or the LP01 mode, if we approximate the VCSEL cavity as a cylindrical waveguide). In the weakly guided core/cladding waveguide, the fundamental mode peaks in the centre of the waveguide, while the higher-order modes are concentrated near the periphery. As a result, the small top aperture reduces the quality factor of higher-order modes, thereby altering the modal characteristics and ensuring that only the fundamental mode is favoured.
We have undertaken DC characterisation of our integrated-mode-select-filter VCSELs at room temperature, using fibre-optic coupling with a ball lens (see Figure 2 for the typical measured optical spectrum). We have found that the fibre-coupled optical power of our lasers ranges from 1.3 mW to 1.6 mW at 8 mA, and the threshold current varies from 0.8 mA to 1.0 mA among all measured devices. All the VCSELs we have characterised exhibit single-mode operation with a fundamental mode wavelength near 852 nm, and have a single-mode-suppression-ratio exceeding 34 dB at 8 mA, satisfying the specifications of the IEEE 802.3 standard.
VCSEL free-space optical coupling setup for long-distance optical fiber data transmission measurement.
To evaluate the variations from one device to another, we have mapped our single-mode VCSELs driven at 8 mA, considering a single quadrant on the 1.2 cm x 1.2 cm sample (see Figure 3 (a), which includes green labels on this map that signify those devices that underwent rigorous examination). We are encouraged by finding that all our devices demonstrate single-mode operation up to thermal rollover.
We have subjected these devices to rigorous testing under different conditions, spanning room temperature to 70 °C and using fibre spool lengths from back-to-back to 2 km. Notably, throughout these examinations, we did not uncover any sign of optical power decline or any shift in the lasing threshold.
Figure 3. (a) The single-mode VCSEL testing map of one quadrant on a 1.2
x 1.2 cm² sample. All tested VCSELs are labelled green, showing
single-mode operation with a side-mode suppression ratio of more than 34
dB. The red dot labelled single-mode VCSEL under test for both DC and
PAM-4 data transmission. (b) The scanning electron microscopy top-view
image of the single-mode VCSEL under test, providing clear
visualisations of the integrated-mode-select-filter mesa structure, the
top p-contact, and the coplanar waveguide used in the high-speed VCSEL
layout. The red dot shows the device location on the wafer map.
Cranking up the speed
Back in 2021, using an early generation of our novel VCSELs, we reported a record speed performance of 38 Gbit/s over 1 km of single-mode fibre, for error-free optical data transmission using a non-return-to-zero configuration. To realise even higher speeds, we can switch to advanced modulation schemes, thanks to the single-mode output of our devices.
One common advanced modulation scheme is Pulse Amplitude Modulation-4. Due to the rapid expansion of cloud services and data centres, this form of modulation has been standardised for 400 Gbit/s Ethernet in the IEEE Standard 802.3. Using Pulse Amplitude Modulation-4, last year we reported 96 Gbit/s data transmission over 1 km of fibre at room temperature.
We have measured room-temperature eye diagrams with this form of modulation, which enables data rates as high as 108 Gbit/s (see Figure 4 for details).
Given concerns related to carbon footprints, another key metric is energy per bit consumption. For 330 m and 1 km links, this is calculated to be 175.1 fJ per bit and 196.9 fJ per bit, respectively.
Figure 4. Single-mode VCSEL PAM-4 eye diagrams at (a) 108 Gbit/s (300 m
SMF-28) and (b) 96 Gbit/s (1 km SMF-28) with a standard 4-tap
feed-forward equalizer. The device under test was supplied with 7 mA DC
current via SHF BT65R bias tee and directly modulated by the grey-coded
PRBS13 (PRBS13Q) data pattern, which is generated by the Keysight 120
GSa/s M8194A arbitrary waveform generator. All eye diagrams are
un-averaged, and the transmitter dispersion eye closure quadrature
(TDECQ) value is calculated based on the PRBS13Q pattern. The TDECQ
values were calculated assuming a target symbol error rate of 4.8 x
10-4.
The very promising performance of our directly-modulated single-mode VCSELs makes them compelling candidates for playing a vital role in optical fiber communication, especially in scenarios where production scalability, low manufacturing cost, high-speed and long-distance are essential. Their performance advantages and low cost make them strong contenders for deployment in 400 GBase-DR4 optical links in data centers. We will contiunue to refine their characteristics, with efforts directed at optimising device topology, so this will ensure an even higher reliability and intrinsic device bandwidth for data rates beyond 120 Gbit/s per lane.
We acknowledge the sponsorship of CNICE on the single-mode VCSEL research project by Foxconn Interconnect Technology, a leading interconnect company led by Mr. Sidney Lu, UIUC’s distinguished alumni. The authors would also like to thank Dr. Mike Gerhold for ARO support for Microcavity Laser Design and PAM-4 Test under. No. W911NF-22-1-0046.