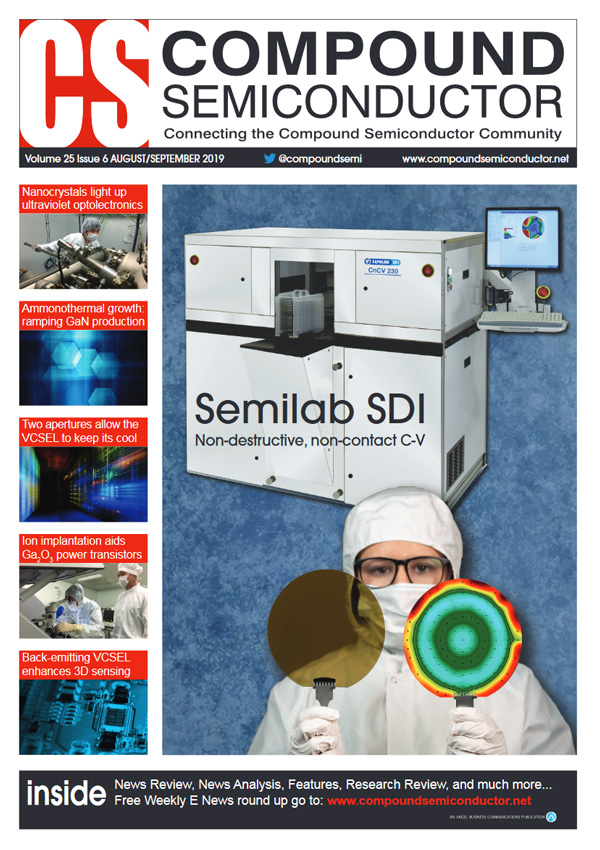
5G: Evolution then revolution
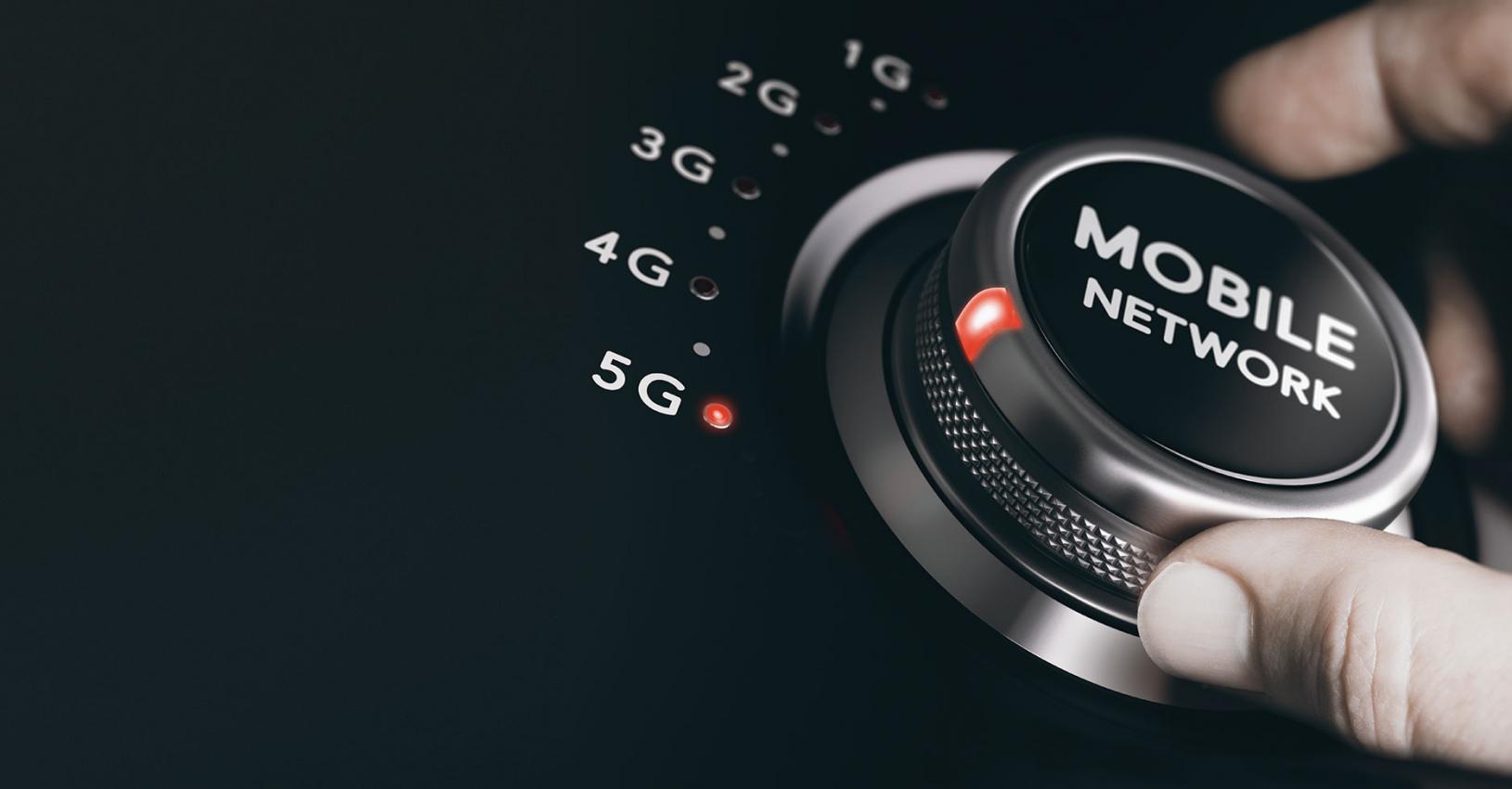
The roll-out of 5G is going to benefit the compound semiconductor industry. Volumes of GaAs HBTs and GaN HEMTs are set to flourish, and a ramp in other technologies could follow, such as that of the GaAs pHEMT and the GaN-on-silicon transistor
BY RICHARD STEVENSON
There are some people that always want the latest and the best. They are sure to be ones to splash out on a 5G smartphone, allured by the promise of a faster connection that could enable a 4K movie to be downloaded in a minute or so.
It is likely that these early adopters will be heavily disappointed. If they live in a city that has just rolled out 5G, it is true that they will enjoy better connectivity – and when everyone wants to access the internet at the same time, that’s highly valued. But if they expect to download data at breath-taking speeds, they will probably be in for a shock. According to many experts, the increase in speed will be only about 20 percent.
Why are these expectations going to be so different from reality? Well, it’s because 5G is being deployed in two phases – and it is only the second phase, which doesn’t start rolling out until around 2023 at the very earliest, that is going to deliver a substantial hike in data rates, thanks to a move to wider frequency bands in the millimetre-wave spectrum above 24 GHz.
Right now, it is only the roll-out of a sub 6 GHz band that is underway. This typically operates at a frequency of 2.6 GHz or more, and as well as increasing coverage for subscribers, it will open up new business opportunities for operators, such as industrial monitoring.
Only by understanding the details of this two-phase deployment, as the analysts at Yole Développement do, is it possible to predict how the emergence of 5G will impact sales of RF compound semiconductor devices. According to experts at Yole, the roll-out of 5G will be good for GaAs and GaN. Production of GaAs-based power amplifiers for mobile phones will increase, as the introduction of the new network will lead to, on average, an additional amplifier in every phone; and sales of GaN HEMTs will rise as carriers deploy 5G infrastructure, which will start forming national networks in the next few years.
In cities in South Korea, the UK, the US, Japan and China, deployment of a 5G, sub 6 GHz network is already underway.
“The technology looks a lot like LTE,” says Antoine Bonnabel, Technology & Market Analyst for the Power & Wireless team at Yole. Thanks to this similarity, Bonnabel argues that it is relatively easy to develop and implement this form of 5G, with existing base stations upgraded to include this new communication technology.
In some dense urban areas, operators are improving their coverage by also introducing a network of small cells. This has happened in South Korea, where Samsung has deployed 53,000 of them, operating at 3.5 GHz. Operators are using these cells to initially provide LTE coverage, but in time they will be upgraded to operate on the 5G network.
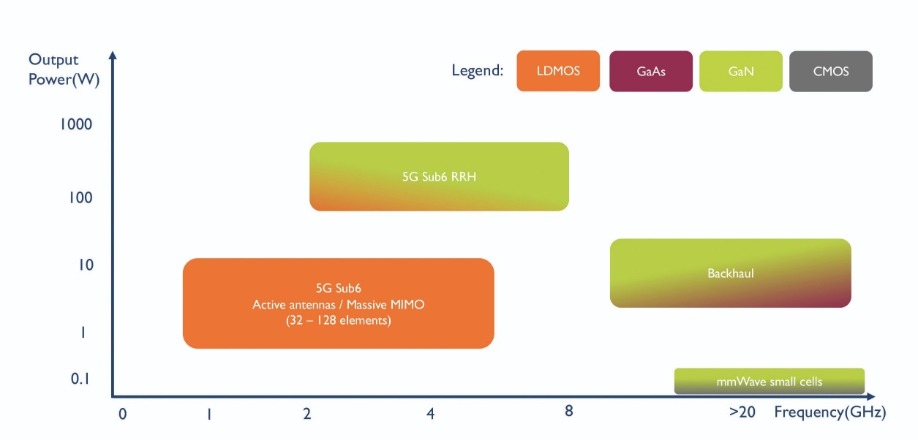
Today, the two most common technologies for wireless communication are silicon LDMOS and GaN, with the latter having the upper hand at higher frequencies. Taken from 5G Impact on Telecom Infrastructure report, Yole Développement, 2019.
Small cells are also being deployed in large numbers in the US. In 2018, Sprint added 27,000 small cells, spread across nine cities. These cells operate at 2.5 GHz.The output of these small cells is less than that of conventional base stations. Rather than producing 240 W, it’s typically between 40 W and 80 W. “It’s still high power,’ says Bonnabel. “You can reach up to a few hundred metres.”
GaN versus silicon
For many years, network operators had to choose between silicon LDMOS and GaN to provide the amplification in the base station. For the emerging 5G networks, the base station needs to deliver a high-power at a relatively high efficiency, a requirement that plays into the hands of GaN. According to Bonnabel, for all the 5G base stations built with conventional technology, as frequencies are above 2.6 GHz, GaN is the only viable option.
As carriers have moved from one generation of communication technology to another, they have also, independently, advanced their antennae technologies. One innovation has been the introduction of active antennae, a move that allows beam-forming and massive MIMO. To deliver these technologies, a small front-end is employed at each antenna, rather than a single front-end for all antenna elements. Configuring the base station in this manner slashes the power requirement for each antenna element, opening the door to the use of silicon LDMOS in 5G networks.
However, even though GaN faces competition from silicon LDMOS for some tasks within the 5G network, shipments of this device will still rise fast. According to Bonnabel, sales of GaN devices to the base station market will increase from $300 million this year to $750 million by 2024.
Bonnabel says that today, the only substrate used for the production of these GaN HEMTs is SiC. However, he points out that the opportunity to turn to a silicon substrate has attracted much interest within industry, due to its potential for low cost, with prices approaching those of silicon LDMOS.
Grabbing the lion’s share of the GaN-on-SiC market is the Japanese firm Sumitomo, which ships its GaN amplifiers in the form of discrete devices. “The main reason [for their dominance] is that they are the exclusive supplier of Huawei,” says Bonnabel, who believes that Sumitomo’s success is deserved, because it has the best technology – and it continues to refine it. To cater to the growing market, Sumitomo is increasing its production capacity, a move that will help it to stay ahead of a chasing pack that includes Wolfspeed and Qorvo. Legislation in several countries, related to security concerns, has dented Huawei’s attempts to win contracts to deploy 5G all over the world.
“China, because of this ban, has invested more in its 5G deployment than it was supposed to,” says Bonnabel. “The investment that they were supposed to make over the course of three years, they have made it in a year.”
One advantage that Huawei has over its peers in the 5G market is that it has a lot of expertise in making and deploying devices operating at relatively high-frequencies within the sub 6 GHz band. In comparison, the main frequencies in today’s networks in the US and Europe are below 2.6 GHz, a domain well served by Nokia and Ericson.
Ironically, it is only recently that the US has permitted the use of higher frequency bands, such as those at 3.5 GHz and 4.2 GHz. In developing countries, legislators are far more willing to permit the use of higher frequencies, and operators are using them for 4G deployments. “They go for Huawei products, because they are 30 percent cheaper than Ericson,” says Bonnabel.
5G smartphones
Alongside the roll out of 5G networks is the introduction of a handful of compatible smartphones. Aside from a couple of exceptions, 5G capability is limited to the sub 6 GHz network.
So far, 5G smartphone sales have totalled between 5 million and 10 million, according to Cédric Malaquin, a Technology & Market Analyst who specialises in RF devices & technologies and works within the Power & Wireless division at Yole. Malaquin expects that even by the end of this year, sales of 5G phones will not have exceeded 50 million, and it will take until 2025 before they can account for 30 percent of the market. “So, in number, something like 600 million units.”
The compound semiconductor content in the smartphone is in the form of a GaAs power amplifier. “The power and efficiency is unmatched by any other technology, especially CMOS,” says Malaquin. He expects the dominance of the GaAs power amplifier to continue, as operators are requesting more and more power from the device to the base station.
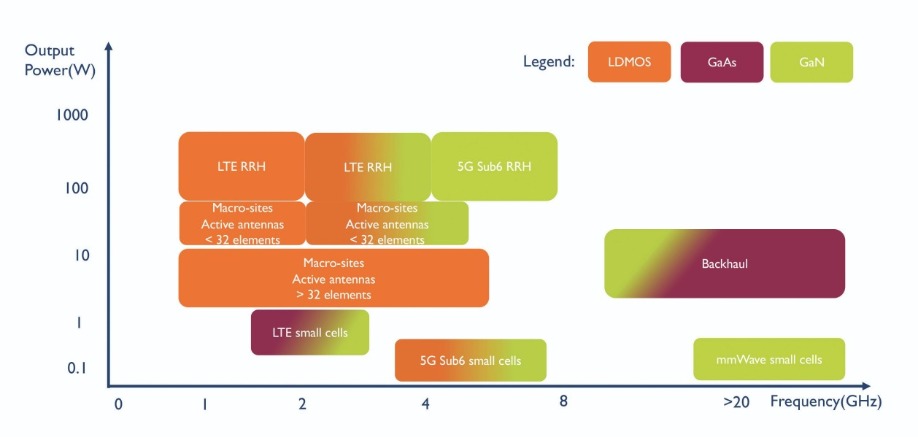
By 2025, GaN will be the dominant technology in traditional base stations, and in millimetre-wave 5G. However, in massive MIMO, silicon LDMOS is tipped for success. Taken from 5G Impact on Telecom Infrastructure report, Yole Développement, 2019.
Rewind the clock a few years and manufacturers of GaAs power amplifiers, such as the big four from the US – RFMD, Skyworks, TriQuint and Anadigics – would be striving to increase their sales of discrete devices. But that’s no longer the case. The battleground has changed, with competition now surrounding sales of a front-end module, which incorporates a GaAs power amplifier, along with a filter and a chip that switches between the frequency bands.Rivals in this sector include Skyworks, Murata and Qorvo – an entity formed from the merger of RFMD and TriQuint – that are drawing on their in-house GaAs power amplifier technology to make front-end modules. Up against them are the likes of Qualcomm and Broadcom, all making their own GaAs power amplifiers by drawing on the services of GaAs foundries, such as WIN Semiconductors of Taiwan.
It is not easy to assess the impact of the introduction of sub 6 GHz 5G networks on the production volumes of GaAs power amplifiers. In some countries, such as Japan, the established 4G networks operate at high frequencies, and it is possible that a design engineer could use one amplifier for both the 4G and 5G signals. But that is not the norm, according to Malaquin, who has calculated that, on average, the introduction of 5G will lead to the addition of one power amplifier per phone. That’s good news for the makers of MOCVD tools, GaAs substrates, metal-organics sources and characterisation equipment, that will all welcome the additional business.
Note that the key component in the front-end module is not the GaAs power amplifier, as its production can be outsourced. “It’s more important to have the right cost and the right performance for the filter,” says Malaquin, who argues that it is the excellence in this technology that is behind the success of Skyworks, Qorvo, Broadcom, Murata and Qualcomm.
Millimetre-wave networks...
The second phase the 5G rollout – the introduction of a millimetre-wave network – will require a revolution, rather than just an evolution, in infrastructure. At frequencies of 24 GHz and above, signal attenuation is far higher, so the smartphone must be far closer to the base-station. Consequently, there needs to be a far denser network, which will be made of small cells. Deploying this only makes sense in areas where there are large crowds, such as sports stadia.
Trials of millimetre-wave networks are underway. In the US, there has been great interest in this technology, but according to Bonnabel, those working in this area have found it more challenging than expected. “Right now there is a move back from millimetre wave, and larger interest in sub 6 GHz frequencies.”
Bonnabel believes that it will be 2023 before the millimetre-wave market starts to develop, and from then on growth will be modest. “Operators spend a limited amount of money on their annual deployment, so the amount of money for millimetre-wave small cells will be limited, and will not grow,” reasons Bonnabel. He expects a saturation in sales to the small cell market to occur by 2025. “We don’t expect it to be a booming market that will see millions and millions of devices.”
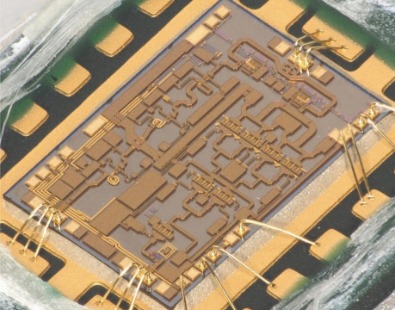
An optical image of a Qorvo 39 GHz GaN-on-SiC MMIC front-end module that is targeting 39 GHz phase-array base stations and terminals.
It is not yet clear what technology will be used in these small cell deployments. In today’s trials, millimetre-wave cells employ GaN. “In the longer term, when you reach a large number of antenna per small cell, you may not choose a power amplifier, and you might be able to use CMOS itself,” argues Bonnabel.
…and smartphones
For those living or working where 5G millimetre-wave trials are taking place, there is the opportunity to experience this revolutionary network by buying one of a very small number of compatible handsets. They include a version of the Samsung SG105G, and a solution from Motorola. It far from easy to cram the additional technology into a phone that enables it to operate in the millimetre-wave band. In fact, Motorola doesn’t even try, preferring instead to have a separate unit for this spectral domain, and strapping it onto one of its Z-Series phones (see the image on the right).
Designing a handset that works well at these frequencies is a tough ask. Antenna gain is low, and current CMOS power amplifiers are inefficient. “It’s a problem for your battery life,” says Malaquin.
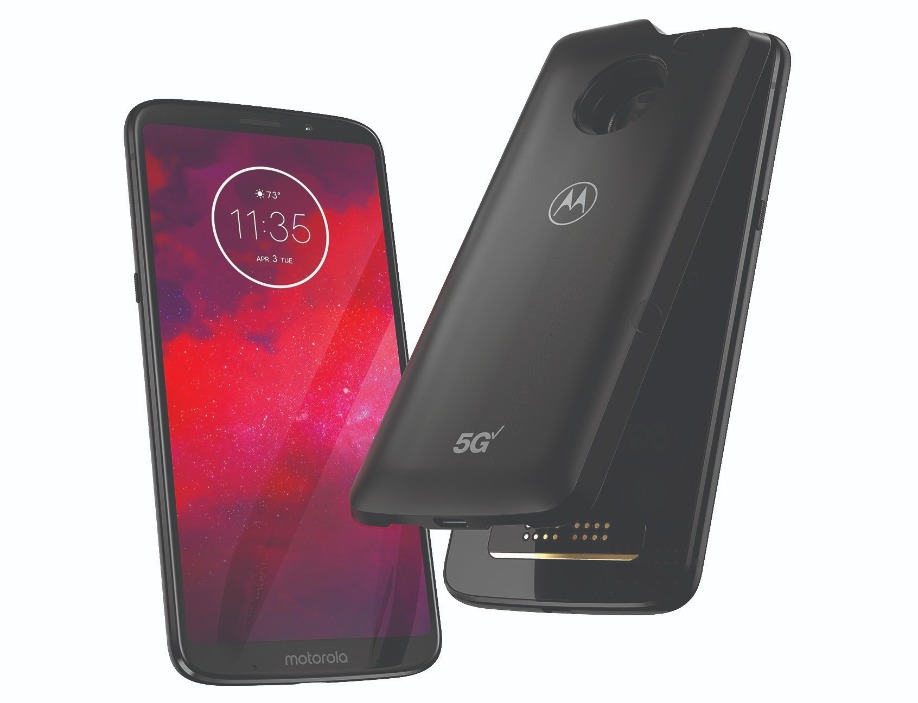
The 5G Moto Mod, which provides millimetre-wave connectivity, places a 5G modem inside a plastic backpack. Using magnets and pogo pins, this unit bonds to a Motorola Z-series phone.
A teardown of the Motorola millimetre-wave unit shows that it employs a silicon-based power amplifier. “The efficiency is quite poor,” says Malaquin, who believes that engineers adopted this approach because it is the quickest way to get to market.Over the next few years, other technologies could come to the fore. “Above 6 GHz, your HBT today doesn’t do the job,” says Malaquin. “But the pHEMT, it’s a different story – you can use the pHEMT design to do a millimetre-wave power amplifier.” Another candidate is GaN-on-silicon, a technology dogged by concerns over reliability.
This is further evidence that there is much uncertainty surrounding the revolutionary phase of 5G. That’s certainly not the case for the first phase, which is an evolution of 4G LTE that will drive an increase in the production of GaAs HBTs and GaN HEMTs. So, in the short term, 5G is definitely going to be good for the compound semiconductor industry – and looking further ahead, there could be additional sales from the roll out of millimetre-wave networks.
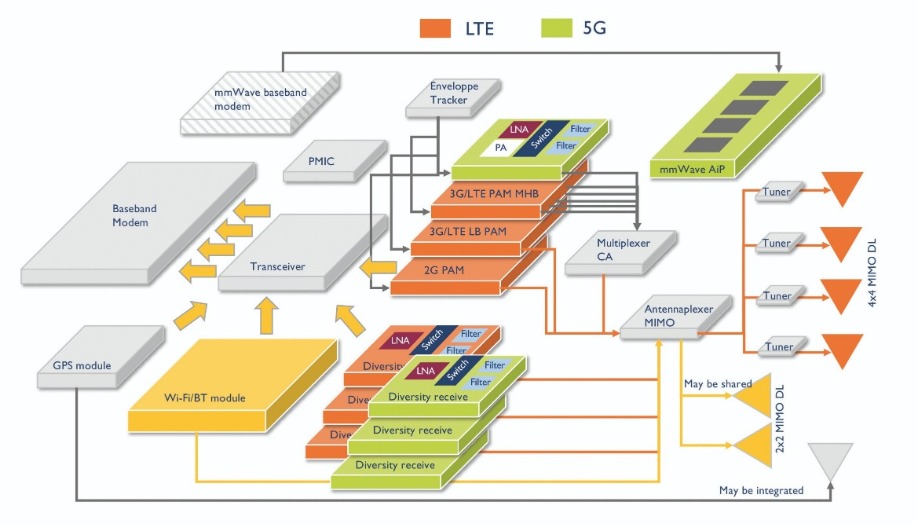
The introduction of 5G will have an impact on the architecture of the RF front-end of mobile devices. Taken from 5G’s Impact on RF Front-End Module and Connectivity for Cell phones report, Yole Développement, 2019.