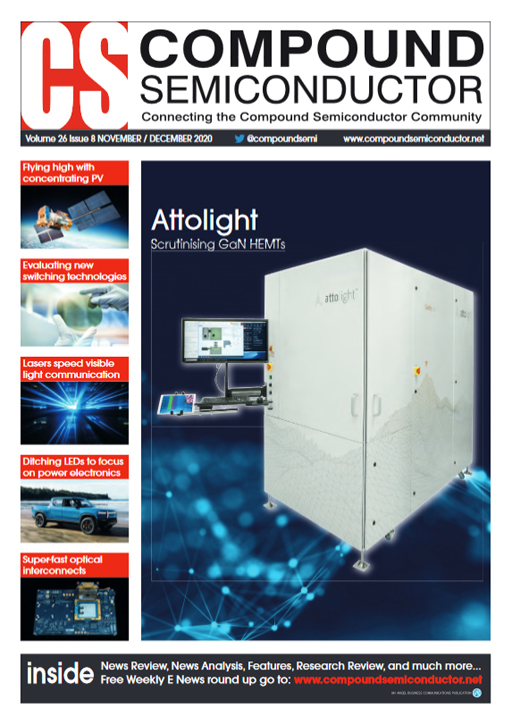
Lasers: Accelerating visible light communication
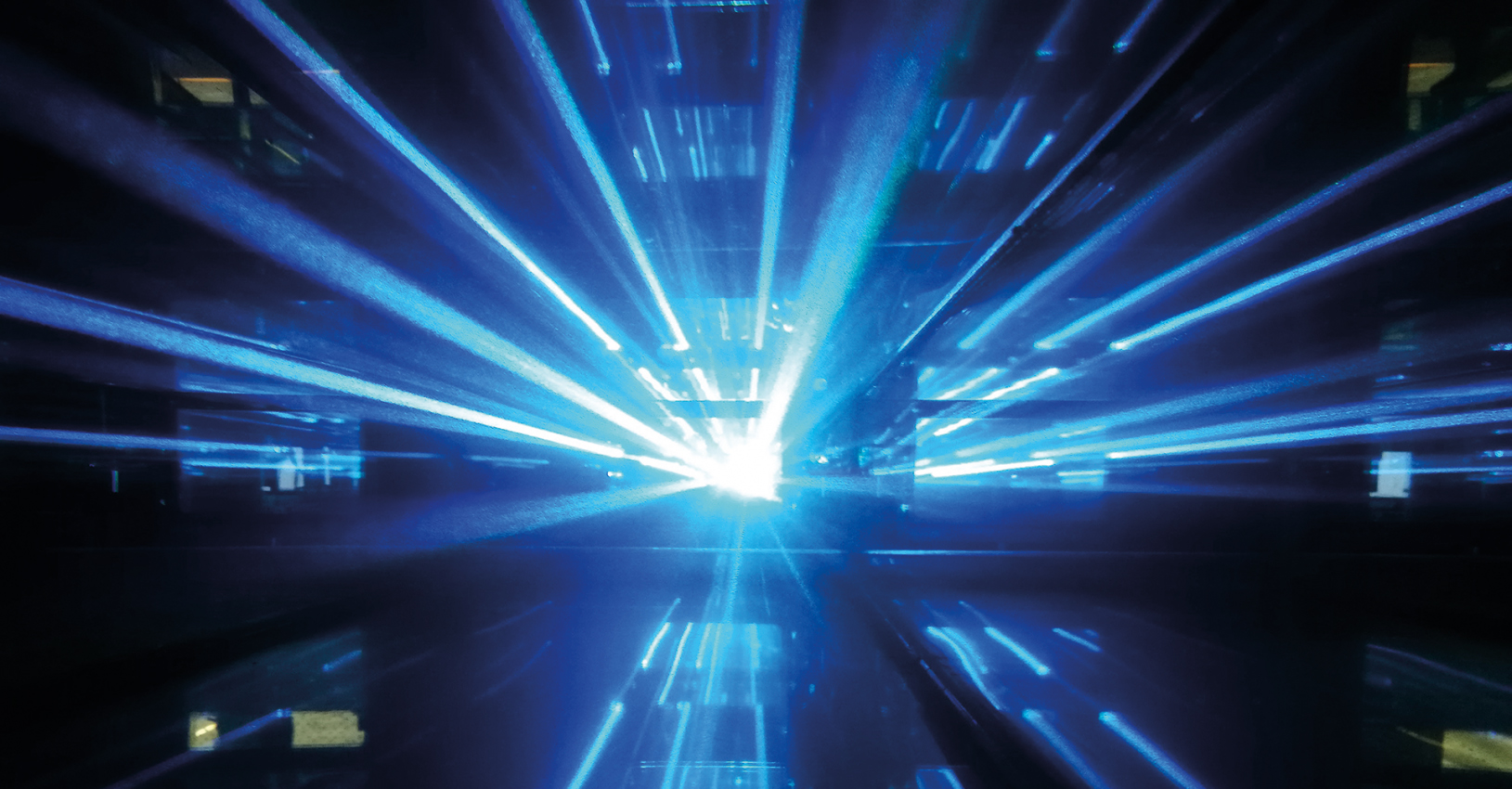
GaN-based single-mode lasers get ready to revolutionise communication
BY MEIWEI KONG, JORGE HOLGUIN-LERMA, TIEN KHEE NG AND BOON S. OOI FROM KING ABDULLAH UNIVERSITY OF SCIENCE AND TECHNOLOGY
THERE IS GREAT DEAL going for the use of light for wireless communication. Compared with the standard radio frequency bands employed by mobile devices, the bandwidth is unlicensed and more than a thousand times greater. However, despite these merits, it’s not to say that it has to be one technology or the other. Far better is a future that sees light-based wireless communication merge with existing technologies operating in the radio and millimetre-wave regime to spawn a synergic scheme that drives a new era for connectivity.
While the light source for communication can operate in the UV or the IR, it is far better if it is in the visible, as this allows simultaneous illumination and communication. Communicating via visible light is already attracting much commercial interest, thanks to its capability to transmit data at high speeds, its compatibility with large-scale integration, and its freedom from electromagnetic interference.
The conventional light source for visible light communication is the LED. This device is normally in the form of a white emitter, created by coating a blue GaN-based chip with a colour-converting phosphor. Such devices have fuelled the rise of visible light communication, and offered a vision of street lamps providing internet to anyone passing by.
By adopting advanced modulation technologies, researchers have been able to coax data rates of up to several Gbit/s from a single LED. Increased speeds and greater modulation bandwidth have come from miniaturising the device to form a microLED. However, faster speeds come at the expense of output power. Select a conventional LED and you’ll get a high optical power and a brightness well-suited for illumination, but the modulation speed is limited; replace this with a microLED and you’ll enjoy a very attractive modulation speed, but you’ll fall short in illumination.
Fortunately, there is an alternative that eliminates compromise: the laser diode. But is it a good solution? Absolutely, according to our team at the King Abdullah University of Science and Technology. We have been building on the efforts of developers of lasers based on distributed feedback (DFB). These devices can combine lighting levels of brightness with data rates that are higher than those of microLEDs.
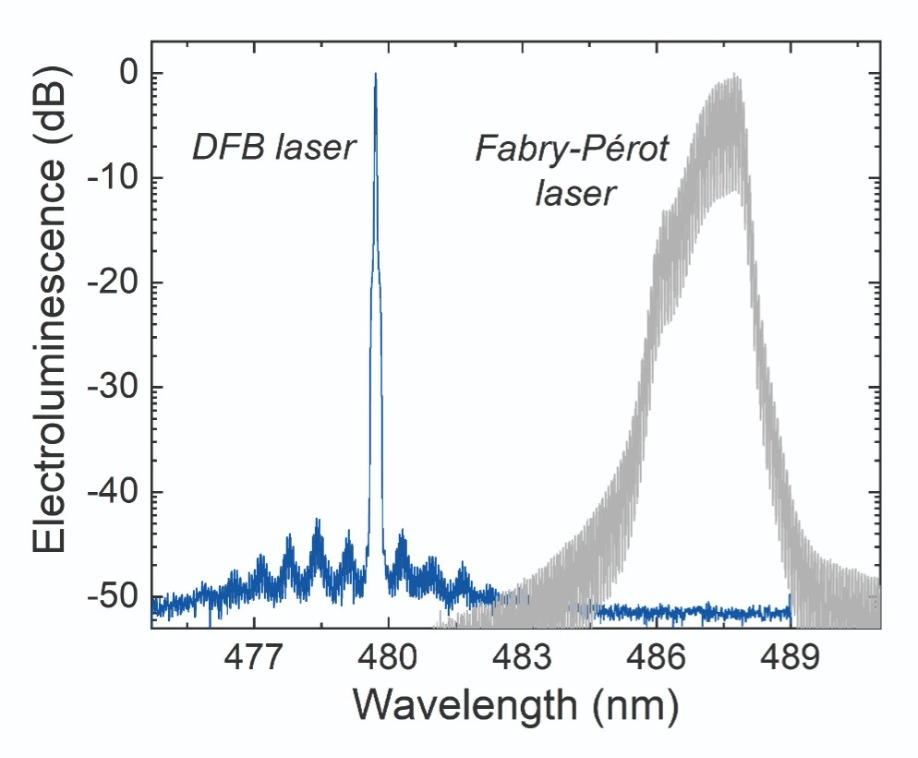
Figure 1. The multiple cavity modes of a Fabry-Pérot laser can be reduced to a single mode by adding a DFB grating. The figure shows a GaN-based sky-blue colour laser.
Transmitting data wirelessly at high speeds is in much demand. It is needed for emerging applications that include virtual reality, augmented reality, and autonomous driving. This demand has led to new ventures, such as laser-interconnected satellite constellations, such as Starlink, developed by SpaceX. Efforts are also directed at constructing next-generation communication networks known as LiFi. In all these cases, the goal involves establishing a ubiquitous wireless network, unbounded by the limitations of classic infrastructure. The vision is clear; it will not be long before wireless data is burdened by a congested radio-frequency spectrum. Light-based wireless communications in the IR, visible and UV offer a solution, and could make a valuable contribution to 5G networks.Laser credentials
Laser diodes are well-positioned to make an impact. These energy-efficient sources combine high-brightness illumination with very high-speed data transmissions, and have the potential to replace LEDs in very-high-speed visible light communication applications, such as smart factories, intelligent transportation and large-capacity broadcasting, where data rates need to be at least 10 Gbit/s. Highlighting what is possible are the white-light sources containing blue GaN lasers, which are now appearing in the market, launched by companies such as SaNoor Technologies and SLD Laser. Variants have also been deployed in cars, with laser headlights powered by Osram’s chips available in some models from BMW and Audi. And if you take a glance at the scientific literature, you’ll see many reports on the impressive properties of lasers for record-breaking visible light communication, leading one to wonder what more is to be done.
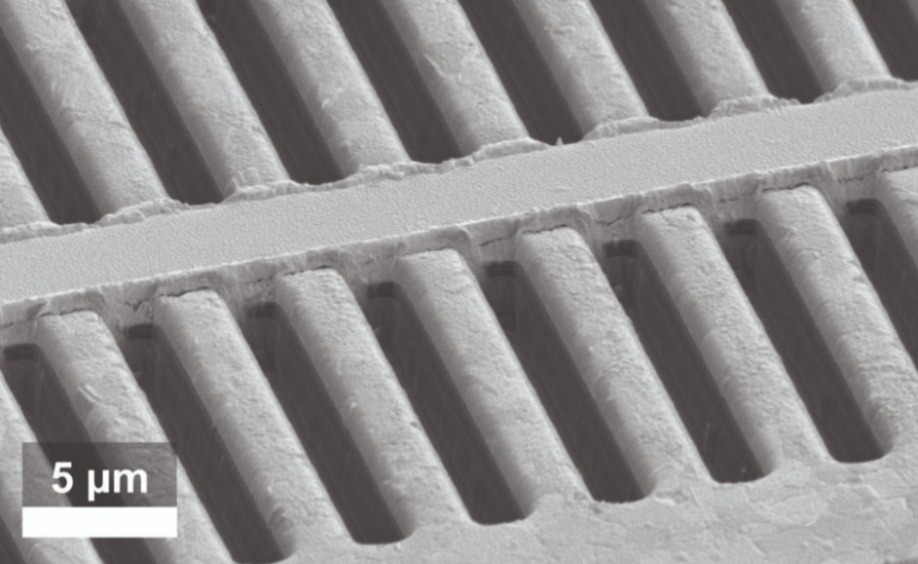
Figure 2. A DFB grating fabricated on the surface of a laser diode from KAUST. Using a surface grating allows a single-mode output without the need for the difficult overgrowth process in GaN lasers.
One route that we are pursuing, which can lead to far higher date rates than have been seen to date, is to switch to a different laser architecture. Conventional GaN laser diodes feature a Fabry-Pérot cavity that gives rise to longitudinal modes known as Fabry-Pérot cavity modes. These modes, which lead to the emission of a range of closely spaced wavelengths, are fairly acceptable for many applications. However, this architecture is not used in the telecommunication industry, which employs wavelength-division multiplexing to increase the data transmission capacity of optical fibres by a factor of several hundred. The key ingredient for wavelength multiplexing is the single-frequency or single-mode laser. This is the way to go for visible light communication.It is possible to produce a single-frequency source with a solitary longitudinal mode by modifying the Fabry-Pérot laser (illustrated in Figure 1). Single-mode operation can be achieved in several ways. One can introduce external elements and external laser cavities, such as prisms, gratings, and mirrors mounted on an optical bench. But it is preferable to avoid moving parts, and create instead a compact source that is immune to vibrations, with the technology for realising a single mode self-contained on a single chip. This type of device, sporting a monolithically integrated distributed-feedback (DFB) grating, uses diffraction to ensure feedback into the laser cavity at a specific frequency. The frequency that becomes the dominant mode is determined by both the material used in the laser and the geometry of the grating.
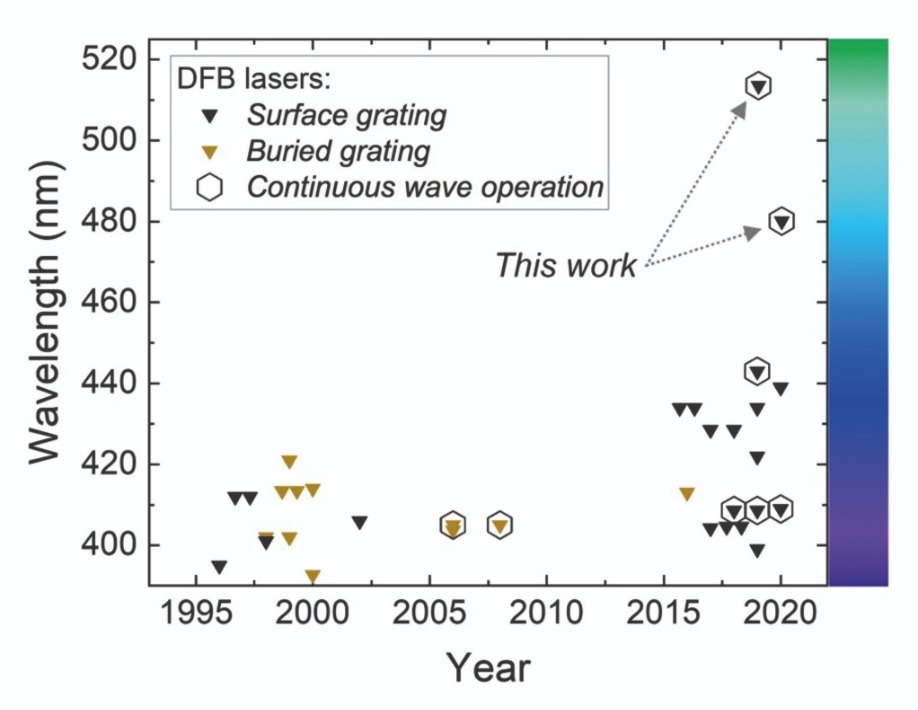
Figure 3. The history of GaN-based DFB lasers at different wavelengths with the rise of surface gratings, including KAUST’s contribution to unexplored wavelengths in the blue-to-green regime.
InP-based DFB lasers are widely available at telecommunication wavelengths, but in the visible spectrum, especially in the blue and green – a domain only reached using GaN materials – they are relatively unexplored. In fact, there are no commercially available GaN-based DFB lasers.The history of the DFB laser can be traced back to 1996, just a year after the device made its debut. Researchers at the University of Stuttgart, Germany, are to thank for this breaking of new ground – but their aim was not to realise single-mode lasing. Instead, they introduced DFB to address the poor quality of the mirrors in the laser cavity. Back then, the hardness of GaN and its crystal properties hampered efforts to cleave and etch the cavity mirrors.
Problems associated with making mirrors have been addressed, but difficulties in processing GaN remain. These issues need to be understood in order to appreciate the state-of-the-art methods employed for creating modern GaN DFB lasers.
To produce the classical DFB gratings found in InP lasers, engineers embed the grating inside the device. These buried gratings are formed by growing the initial layers of the laser, before etching and subsequent growth of a second stack of materials – the latter process is known as overgrowth.
Producing gratings by this complex approach is particularly demanding for GaN-based materials. Even though buried gratings were used in the first GaN DFB laser diodes producing continuous-wave operation, announced in 2006 by Nichia Corporation, difficulties in processing and overgrowth still present a significant challenge. Illustrating this point, no further work was reported for ten years.
Surface gratings
A radical re-design is to thank for a new era of GaN DFB lasers. Switching from buried to surface gratings has opened the door to the use of highly mature materials, employed to create the latest generation of compact single-frequency DFB lasers at violet and blue wavelengths (see Figure 2). Unlike buried gratings, surface gratings are simply formed on top of the laser, eliminating the need for overgrowth. This makes them easier to fabricate. Look back at the paper detailing the DFB laser from 1996 and you’ll find that this was the approach taken back then. But only recently have fabrication and material-growth skills fused together in pursuit of creating GaN-based single-frequency lasers.
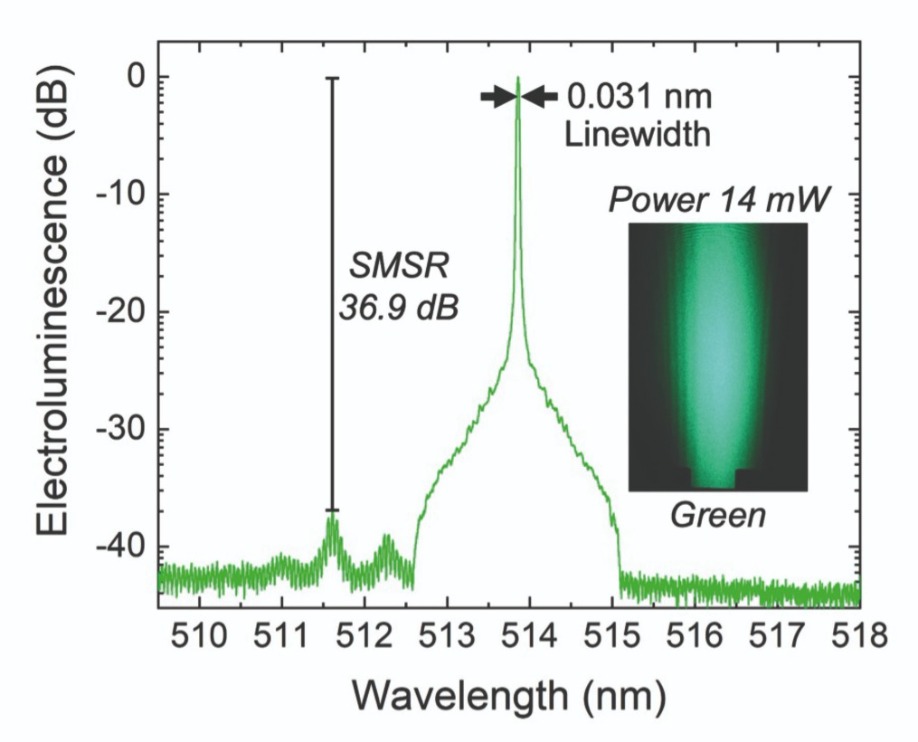
Figure 4. Green DFB laser emission shows a resolution-limited single-mode at around 514 nm. The inset reveals a real-colour image of the laser far-field pattern. The side-mode suppression ratio (SMSR), linewidth, and optical power help to evaluate the quality of the DFB laser.
Uses for these single-frequency visible DFB lasers extend beyond what one might think of as very-high-speed visible light communication. Two examples are miniaturized atomic clocks, which could soon become a reality; and wireless internet underwater, due to the overlap of the blue-to-green spectral domain with the transparency window of ocean and sea waters.To increase data rates underwater, and also above ground and in space, systems can be built that match the emission of the DFB laser to one of the low-intensity wavelengths of sunlight, also known as a Fraunhofer line. This provides a boost to the signal-to-noise ratios of communication channels and environmental sensors. As far back as 1986 researchers at the the Jet Propulsion Laboratory were evaluating the use of Fraunhofer channels for data transmission in space; by 1992 scientists at the US Naval Command, Control and Ocean Surveillance Center were exploring this idea for underwater communications; and in 2013 Ocean University of China reported new observations of improved environmental sensing, using sources operating in Fraunhofer lines.
We are building on this work by investigating visible-light DFB lasers that generate single-frequency emission at unexploited wavelengths in the blue and green – we are evaluating a domain from 450 nm to 520 nm (see Figure 3). Producing laser diodes in this spectral range is not easy because material quality falls, driving down efficiency, as emission is propelled from the violet towards the green by increasing the indium content within key layers of the device. This material limitation helps to explain why, until recently, there have been no GaN DFB lasers operating beyond 450 nm.
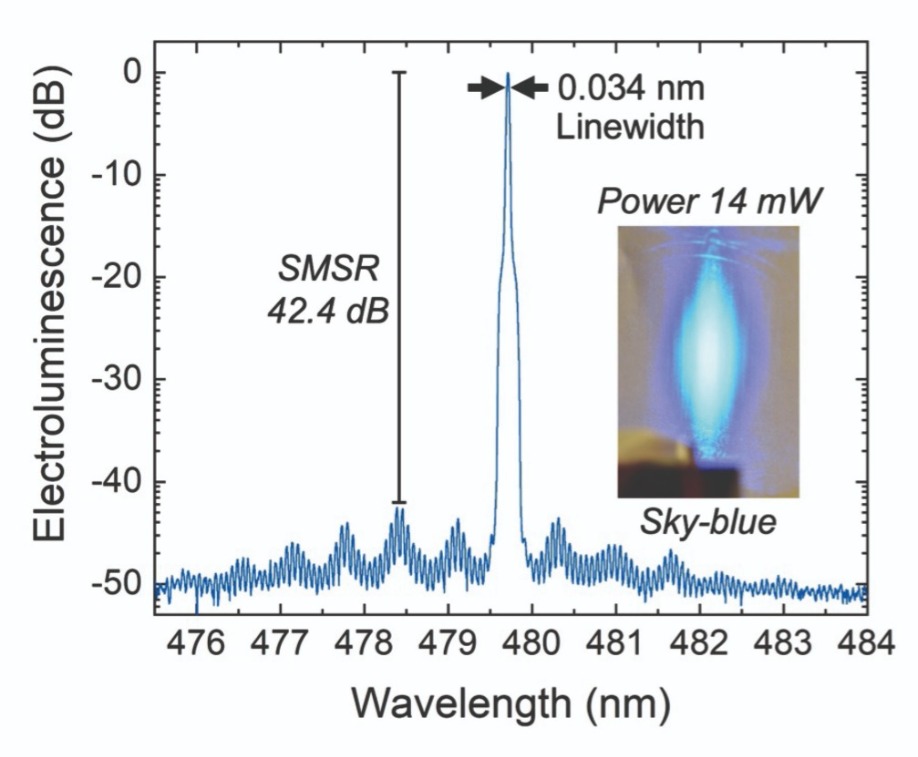
Figure 5. Sky-blue DFB laser emission shows a resolution-limited single-mode at around 480 nm. The inset shows a real-colour image of the laser far-field pattern. The side-mode suppression ratio (SMSR), linewidth, and optical power help to assess the quality of the DFB laser.
To break new ground, we have turned to a microfabrication technique that is suitable for prototyping. We use a focused-ion beam to define a surface grating on the top of laser diodes that are new to the market. These lasers, operating at around 480 nm (sky blue) and 520 nm (green), have much promise for underwater LiFi and low-background noise systems. That’s because they are in the vicinity of Fraunhofer lines, particularly the Hydrogen-beta line at 486.13 nm and the Magnesium-b1 line at 518.36 nm.As the gratings in our lasers are based on the high-order Bragg condition they are spaced relatively far apart, reducing fabrication complexity. If first-order gratings were used for visible lasers, this would require high-end electron-beam lithography, which is relatively expensive and time-consuming.
Although high-order gratings have the potential to introduce diffractive losses, our devices are still able to generate laser light. We have demonstrated a 513.85 nm laser that delivers a 14 mW output at room temperature and has a linewidth of 0.031 nm with a side-mode suppression ratio of 36.9 dB (Figure 4). In comparison, before we introduced the high-order DFB surface grating into the classical multi-mode Fabry-Pérot GaN-based green laser, this device showed a linewidth of 0.540 nm and a side-mode suppression ratio of 0.2 dB. These changes are very promising, with single-mode selectivity improving by a factor of around 5000 and linewidth reducing by nearly 20 times.
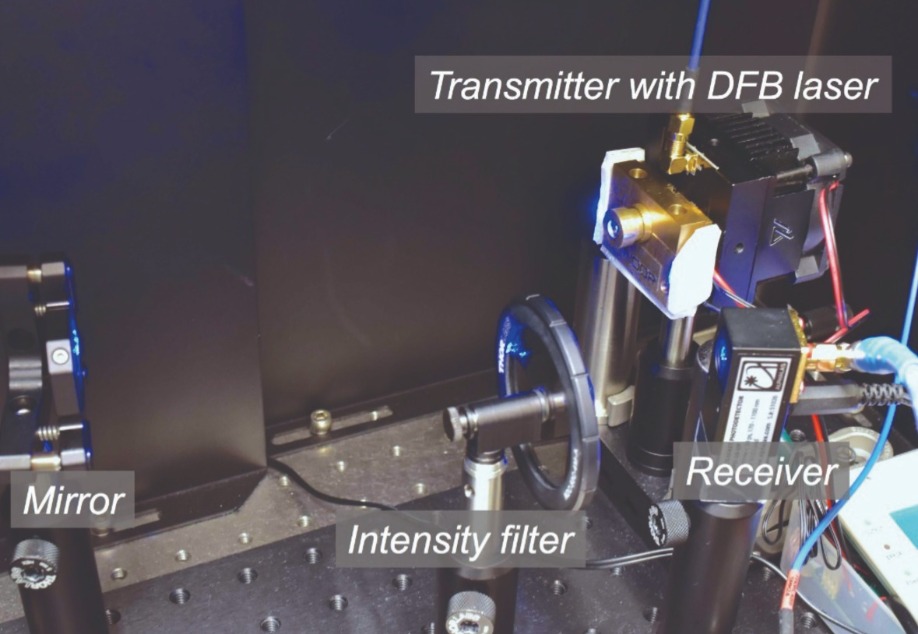
Figure 6. KAUST’s testbed for proof of concept. The goal is beyond 10 Gbit/s using a GaN DFB laser.
We have also produced a variant in the sky-blue with a high-order grating. This 479.7 nm laser produces a continuous-wave optical power of around 14 mW at a slightly cooled room-temperature of 16 °C, has a linewidth of 0.034 nm, and a side-mode suppression ratio of up to 42.4 dB (see Figure 5). This linewidth is narrow enough to enable between 25 and 30 communication channels in the same wavelength window occupied by a single conventional laser, while the side-mode suppression ratio signifies mode selectivity with a factor of around 17,000 – that is, the single-mode of the laser is thousands of times stronger than any other mode in the laser cavity. The superior selectivity of this sky-blue laser compared with its green cousin results from a combination of an optimised fabrication process and a reduction in ridge waveguide width from 4 μm to 2 μm.Building on these successes, we have investigated the capability of our single-mode lasers for visible light communication, evaluating whether they can operate at more than 10 Gbit/s, a requirement for beyond-5G networks. Using a proof-of-concept testbed with minimal components (see Figure 6), we began by considering how fast the laser can be turned on and off. We recorded a relatively flat modulation response for our sky-blue DFB laser, with values for the –3 dB bandwidth and the –10 dB bandwidth of 1.1 GHz and up to 2.6 GHz, respectively (see Figure 7).
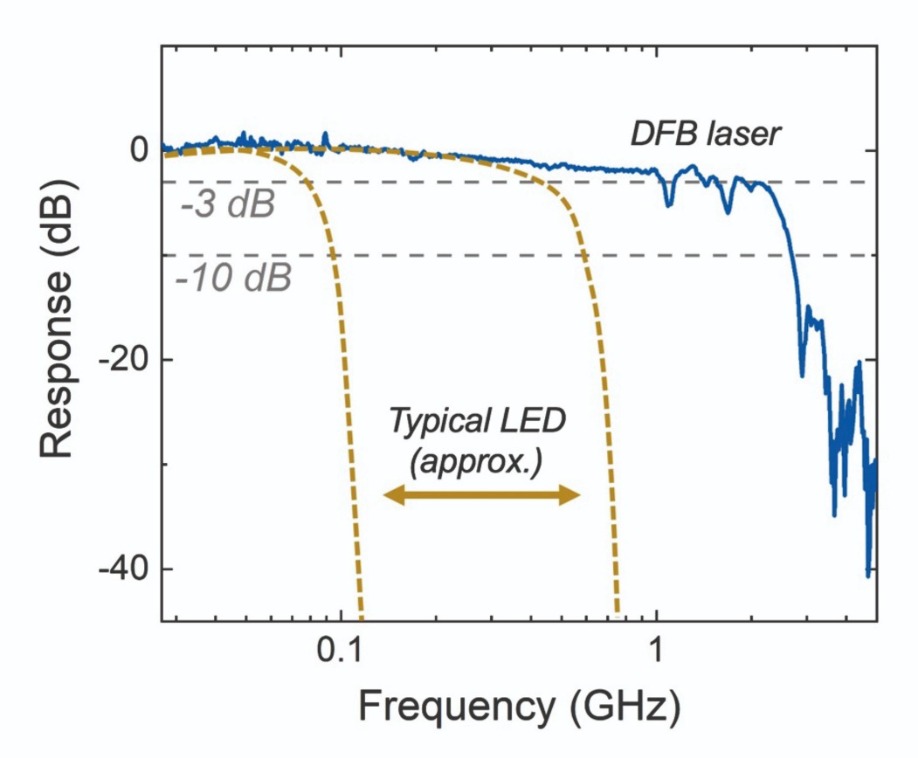
Figure 7. High-speed modulation bandwidth of the sky-blue DFB laser compared with the approximate bandwidth of typical LEDs (ranging from high-power LEDs to faster microLEDs).
These figures indicate that our single-mode lasers offer a significant amount of practical bandwidth for communications. In sharp contrast, a typical LED has a bandwidth of just a few to hundreds of megahertz. Given this substantial gap in performance, it’s of no surprise that lasers are gaining steam in visible light communication.Using a simplistic non-return-to-zero on-off keying modulation technology, our DFB laser realised a transmission speed of 5 Gbit/s. With this form of modulation, on and off states provide the binary information. We visualise the signal that’s received with a plot known as an eye diagram (see Figure 8). In this plot, the upper and lower levels represent the binary nature of the data, and the greater the clarity, the higher the quality of the wireless communication.
To make full use of the modulation bandwidth, we have also turned to a modulation technique called 16-quadrature-amplitude-modulation (QAM) orthogonal frequency-division multiplexing (OFDM). The OFDM technique employs orthogonal and overlapping sub-carriers that adopt the 16-QAM OFDM to improve the spectral efficiency and transmission rate.
We use a constellation diagram to evaluate the quality of the received 16-QAM OFDM signals (see Figure 8). When we see data points that are clearly separated we know that the data has been received with little to no errors. That’s the case for our DFB laser, which provides data transmission up to 10.5 Gbit/s. The associated bit error rate is 2.3×10-3, below the forward-error correction limit of 3.8×10-3, an established criterion in the field of digital communications.

Figure 8. Digital communications can be represented visually depending on their modulation format. The figure shows the eye and constellation diagrams produced by the DFB laser.
This success represents the state-of-the-art for visible DFB lasers for optical communications. But it is only the beginning, providing a small but critical step towards implementing multiple closely adjacent wavelengths. By mimicking the approach adopted in optical fibre communication, we have the potential to multiplex today’s 10 Gbit/s transmission rates by a factor of ten or more.We are now on a journey that could see arrays of single-frequency DFB lasers providing multiple channels of information for wireless internet in underwater sensors and robots, solar-resistant satellite communications, and smart automated factories.
The next step is to scale these results from the level of proof-of-concept up to laser batch-fabrication, which is required for industry adoption. Encouragingly, materials are commercially available with widely accepted process integration, but a stronger market push may be needed.
Another consideration is the verification of the linewidth of GaN DFB lasers. In our work, and in other reports in the scientific literature, optical spectrum analysers are used for this measurement. However, this equipment is limited in its resolution and fails to offer a true telling of the laser linewidth.
Nevertheless, GaN DFB lasers are becoming increasingly mature, and it will not take long before they are deployed in other applications, such as atomic clocks and environmental sensors. What’s more, by targeting Fraunhofer lines, there is the opportunity for new endeavours. Our local measurements show operating at these wavelengths removes a few dBs from background radiation. There are also unexplored DFB wavelengths in the vicinity of the sky-blue and green colour regions that could enhance tools such as bathymetry and underwater optical wireless communications. In addition, narrow-line GaN DFB lasers promise to provide live-cell imaging of Förster resonance energy transfer-based biosensors. Clearly, a great future lies ahead for this visible, single-mode laser.