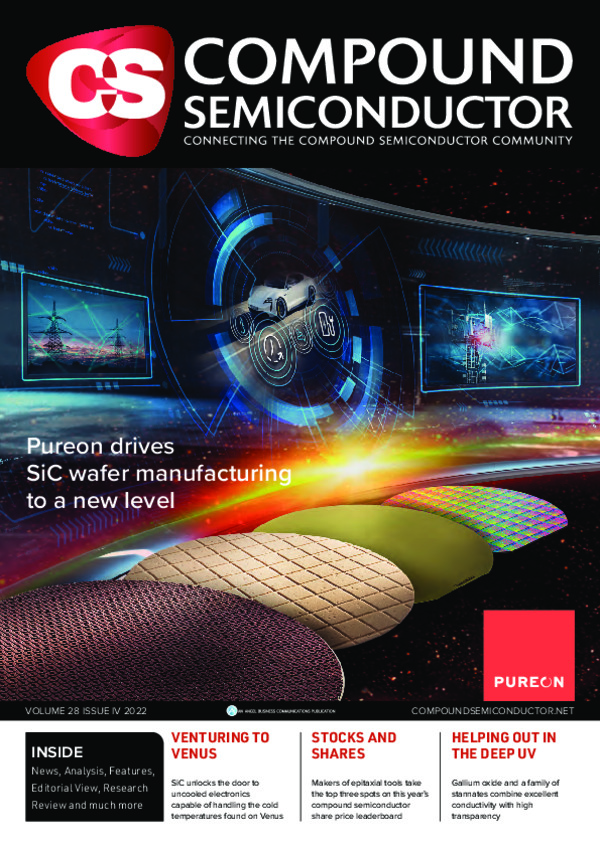
IRPS: Ensuring reliability at elevated frequencies
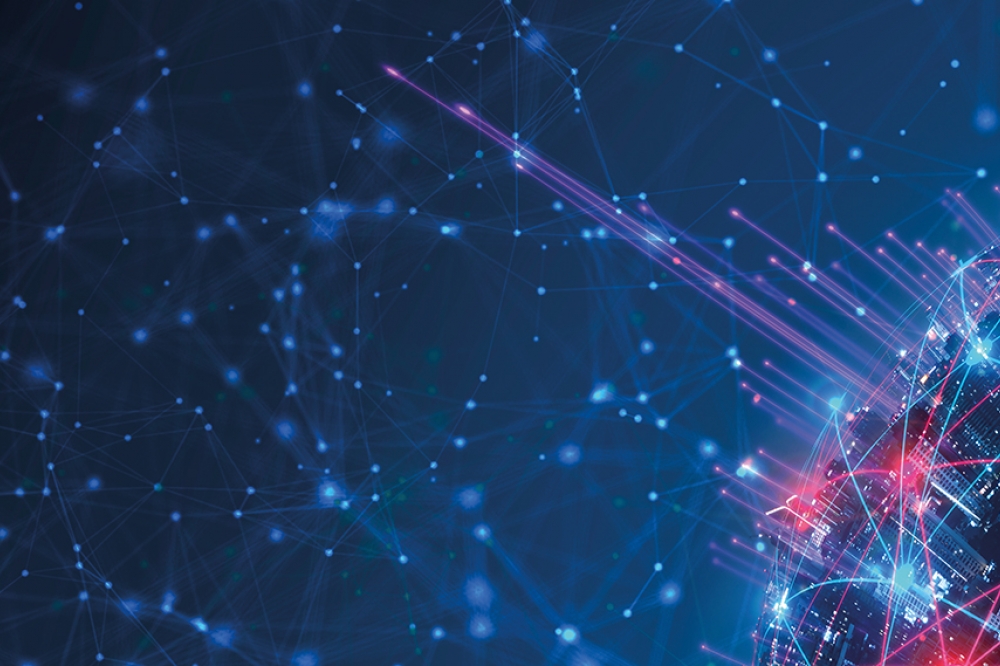
Experimental investigations underscore the opportunities for GaN HEMTs in 5G and 6G networks
BY RICHARD STEVENSON, EDITOR, COMPOUND SEMICONDUCTOR
MANY OF US DREAM of a more powerful car, a bigger TV, a brand-new smartphone or a better pair of speakers. But it’s not failing to own any of them that ruins our day. What irks us is when an item of ours that we really depend upon stops working. When that happens, we are painfully reminded that reliability really matters.
Within the semiconductor industry, reliability is given the high priority that it warrants. Over many years, a great deal of effort has been devoted to analysing and improving the reliability of devices, with much progress reported at the International Reliability Physics Symposium (IRPS).
While presentations at this annual gathering are still dominated by discussions of devices formed from silicon, there is also significant coverage of those made from wider bandgap materials. Last year, we covered presentations at IRPS related to improving the robustness of the SiC MOSFET (see issue IV, pages 32 to 38), and here we report on studies related to the GaN RF HEMT that were presented at this year’s meeting, held in late March. While Covid thwarted an in-person gathering in 2021, this year attendees had the choice of either heading to Dallas, TX, or staying at home and taking part on-line.
The handful of presentations on GaN RF HEMTs highlighted its high power, high efficiency and its ability to operate at high frequencies. Thanks to these attributes, this device is already enjoying much success in mobile infrastructure, as well as defence applications. According to French market analyst Yole Développement, global revenue for the GaN HEMT is undergoing double-digit growth, with sales forecast to surpass $2 billion by 2025.
However, this rosy future should not imply that the GaN HEMT is close to perfection. The reality is that there is much room for improvement. One major issue is charge trapping – this may occur within the barrier, at the interface between this layer and the cap, or in either the channel or the buffer. There are also flaws associated with the use of non-native substrates that lead to dislocations, micro-cracks and warpage of the wafer.
At this year’s IRPS, engineers reported investigations related to a variety of weaknesses associated with the GaN HEMT, and how this could impact its reliability. Those efforts involved studies of devices that can target millimetre-wave 5G, and a discussion of how GaN HEMTs could play a role in 6G, which will require data transfer at far higher frequencies.
Figure 1. For high powers at a high-frequency, GaN-on-SiC is the outstanding candidate.
Targeting millimetre-wave 5G
One of the leading producers of GaN HEMTs for RF applications is the US-based chipmaker Wolfspeed. Speaking on behalf of the company at IRPS 2022, Satyaki Ganguly outlined the capability of Wolfspeed’s GaN HEMTs for sub-6 GHz 5G communications, as well as detailing very encouraging investigations of variants with a shorter gate length that are suitable for millimetre-wave 5G.
For frequencies of just a few gigahertz, several material technologies can be used to produce transistors with sufficient power, including those based on SiGe, silicon LDMOS, GaAs, and GaN built on both silicon and SiC. “But a higher output impedance and a higher power density definitely makes GaN devices more power efficient than others,” argued Ganguly, who claimed that GaN-on-SiC also allows DC power savings when employing massive MIMO and beam-forming technologies.
“Above 6 gigahertz, for millimetre-wave applications, the benefit of GaN is more obvious,” added Ganguly, who pointed out that GaN-on-SiC has a far higher output power at millimetre-wave frequencies (see Figure 1).
Ganguly went on to explain that Wolfspeed’s RF GaN HEMTs are grown on semi-insulating SiC, and feature an AlN barrier and a source-connected field plate. For frequencies below the millimetre-wave, the company offers devices with gate lengths of 0.4 µm and 0.25 µm, with both designs having variants for operation at 28 V, 40 V and 50 V.
Stretching to the millimetre-wave requires a reduction in gate length. This is accomplished with an i-line stepper, employed for producing HEMTs with a 0.15 μm gate length. These transistors, designed to operate at 28 V, have a very impressive set of attributes, including a breakdown voltage in excess of 84 V, a low leakage, a cut-off frequency (fT) of more than 30 GHz, high linearity, an output power of more than 3.5 W/mm, and a power-added efficiency at 30 GHz of more than 30 percent. A three-stage MMIC built with this technology can deliver an output power in excess of 5 W, and operate with a power-added efficiency of more than 30 percent.
Ganguly said that qualification tests on these 0.15 µm HEMTs, produced with what is referred to as a ‘V5 GaN HEMT process’, were a resounding success. No failures were observed during high-temperature reverse-bias tests, DC high-temperature operating life tests, temperature cycles, and RF high-temperature operating-life tests.
The Wolfspeed engineers have also investigated long-term reliability, with RF and DC accelerated-life testing. Defining failure as a drop in output power by more than 1 dB or a catastrophic failure, they determined a mean-time-to-failure of more than a million hours – that’s greater than 100 years – at a junction temperature of 225 °C using RF accelerated life testing. According to Ganguly, a plot of the median-time-to-failure as a function of temperature is similar for devices produced with the G28V5 process and the established G50V3 process, suggesting both these technologies have similar failure modes.
For the DC accelerated lifetime testing, the team defined failure as a 15 percent reduction in the maximum drain current. This suggested a median-time-to-failure of more than 10 million hours at a junction temperature of 225 °C. However, this impressive result must be viewed with some caution, warned Ganguly: “Although the DC result might look attractive, it might not be the most important reliability aspect at normal operating conditions. It gives an overly optimistic prediction.”
Ganguly benchmarked performance against a range of devices provided in a survey by David Via from ARFL. This exercise suggests that Wolfspeed’s HEMTs have an impressive value for median-time-to-failure (see Figure 2). When presenting this data, Ganguly remarked that to the team’s knowledge, it is the first to report reliability RF accelerated life testing data for a gate length as short as 0.15 μm.
Figure 2. Benchmarking the reliability of Wolfspeed’s GaN HENT against
data presented by David Via from AFRL shows that the former has an
impressive level of reliability.
According to Ganguly, over time the specifications for 5G millimetre-wave HEMTs could shift from a junction temperature of 225 °C to 275 °C. Although this would shorten the median-time-to-failure, this might be addressed by introducing the company’s sunken source-connected-field plate design, used to target X-band applications. With that technology, Wolfspeed has demonstrated a saturated output power of more than 10 W/mm, a linear gain in excess of 18 dB, a power-added efficiency beyond 60 percent and a median-time-to-failure of more than 100 years at a junction temperature of 275 °C. These promising figures suggest that even better results are on the horizon for millimetre-wave HEMTs.
Perfecting processing
As one would expect, when different processes are used to produce GaN RF HEMTs, this impacts the carrier trapping within the device, as well as its reliability and performance. A partnership between the University of Padova and UMS has undertaken investigations of this nature, with findings presented at the IRPS by PhD candidate Francesca Chiocchetta.
The portfolio of HEMTs produced by Chiocchetta and colleagues include a control device, formed by using plasma-enhanced CVD to passivate the transistor and CF4 plasma etching to define the gate foot; and variants that employ either low-pressure CVD for passivation, or gate foot etching with a CHF3 plasma.
Investigations of these GaN-on-SiC HEMTs by the European team included high-temperature reverse-bias testing. This considered both gate leakage currents and what is referred to as a ‘belly shape effect’ – this phenomenon, offering an insight into the presence of donor traps between SiN and the GaN cap, is associated with the profile of the gate-source current for small positive gate voltages.
For the device produced with the different form of etching, the results of the high-temperature reverse-bias test included a significant increase in gate leakage, and a belly shape that is ascribed to emission from Coulombic-based traps. “So we can say that the etching variant made with trifluoromethane does not improve the reliability of the device,” reasoned Chiocchetta.
Introducing low-pressure CVD for passivation benefitted the HEMT. It reduced gate leakage and suppressed the belly shape effect. “We can say that we have a better stability of gate characteristics,” argued Chiocchetta. To investigate trapping behaviour in their HEMT portfolio, the team turned to drain-current transient measurements. Plotting drain current as a function of time uncovered two transients associated with the de-trapping process. The etching and passivation processes only influenced the slower transient, which is thought to be related to hopping and surface phenomena.
Chiocchetta revealed that the team is now starting to investigate gate stress prior to breakdown, using electroluminescence to identify defects along the gate finger. It is hoped that after identifying the exact location of the defects within each device, they could undergo a deeper analysis with the likes of transmission electron microscopy. This might expose differences between the reference device, and the passivation and etching variants.
Investigating traps
During the production of RF GaN HEMTs, process engineers introduce iron dopants to the buffer layer to ensure that it is semi-insulating. This step also increases the breakdown voltage, but traps are added that threaten to degrade reliability.
At the University of Modena, researchers have been investigating the influence of iron-traps on the time-dependent voltage of 0.1 µm GaN-on-SiC HEMTs. The team have uncovered a time-dependent occupancy of iron-traps in their devices that they claim can seriously affect the reliability of GaN HEMTs deployed in the upcoming 5G revolution.
The engineers arrived at this conclusion after experimentally evaluating the time-dependent breakdown voltage on scaled GaN HEMTs, and investigating the correlation between the time-dependent breakdown voltage and iron-trap dynamics. Spokesman for the researchers, Marcello Cioni, told delegates at IRPS that the goal of their work had been to evaluate the time-dependent breakdown voltage of GaN HEMTs through pulsed current-voltage characterization, realised by varying the length of the off-state pulse. Efforts involved applying short drain-source voltages to a device in its off-state, and using the drain current to reconstruct the breakdown curve at up to 3 mA/mm, for a range of off-state times.
The engineers have defined the breakdown voltage as that at which the drain current starts to increase vertically. “This is a signature of avalanche generation,” remarked Cioni, who said that for this to occur there needed to be a leakage path and a high electric field.
To identify the cause of the leakage path, Cioni and co-workers measured the drain, gate and source currents for a range of drain-source voltages. Plotting all three currents exposed source-injection as the cause of the device’s breakdown.
By combining simulations with measurements of the drain current at different drain-source voltages, for a range of off-state times, the team concluded that for longer off-state times the trapped electron concentration increased, reducing the free carrier concentration in the high field region. This results in a higher electric field for device breakdown. With shorter off-state times, the concentration of trapped electrons in iron-traps lowers, and the free-electron concentration increases, reducing the electric field required to trigger breakdown. Another impact of shorter pulses is an increase in the avalanche rate, which also leads to a lower breakdown voltage.
Figure 3. For all semiconductor technologies, there is a significant
reduction in the saturated output power with increasing frequency. This
data comes from the Power amplifier performance survey, provided by Hua
Wang and co-workers from Georgia Institute of Technology.
GaN for 6G
Over the next few years the roll-out of 5G will continue. For those that already access this network, their service may not yet include millimetre-wave transmissions, so there is clearly still a long way to go. However, despite much work still to do, some engineering teams are already considering the successor, 6G, which could start to be deployed from around 2030.
Those that are evaluating what form this technology may take, and what role GaN may have to play within it, include Ned Cahoon from GlobalFoundries US. At IRPS he presented details of the capability of 6G, the frequencies it may adopt, and the chip technologies that are best placed for building this next-generation network.
One may wonder whether there is even a need for 6G, given the capability of what we have today. However, Cahoon thinks so, pointing to an insatiable demand for data, along with bandwidth and data rates that are doubling roughly every 18 months. He told delegates that the recent increase in data transfer has been driven by video – this year it has been forecast to account for 79 percent of mobile traffic – while the drivers of tomorrow will be a combination of immersive video and multi-sensory experiences, sensor-driven AI metadata, and holographic telepresence.
“6G promises an order or orders of magnitude improvement over 5G network performance,” remarked Cahoon, who added that it would offer data rates of up to 1 Tbit/s, an ultra-low latency of 0.1 ms, and high accuracy positioning of around 1 cm, enabling joint sensing and communication functions.
To provide all this capability, there is need to use the sub-terahertz domain, taking advantage of the huge amount of available spectrum between 100 GHz and 300 GHz. Within this domain, the corresponding wavelength is around a millimetre or so. That leads to finer resolution radar, which supports the joint communication and sensing objectives of 6G, and enables the use of smaller antenna.
There are challenges associated with the sub-terahertz range. “Losses are much higher above 100 gigahertz,” explained Cahoon, who pointed out that free-space loss increases with the square of the frequency, and in addition the routing loss on and off of the chip is very high.
“More importantly, the fundamental device performance is much worse,” he added, illustrating this point by showing that for all technologies – that includes CMOS, SiGe, GaN, GaAs, LDMOS, InP, oscillators and multipliers – the saturated output power falls with increasing frequency (see Figure 3). “So all of the parameters that are important at the phased-array front-end of the antennae interface get worse – for the PA, the gain output power and efficiency are much worse; for the LNA, the gain and noise figure is much worse – and this directly impacts the transmit output power and the receive sensitivity.” In turn, the link range and data rate are impaired.
Another major issue with increasing frequency, and the corresponding reduction in wavelength, is the reduction in phased-array spacing. Going from a 28 GHz die with an 8 by 8 array to a 300 GHz equivalent slashes the chip size from 47 mm by 47 mm to just 5 mm by 5 mm. As well as a need to scale size while accommodating circuitry in less space, there is a thermal management challenge to overcome.
Fortunately, phased-array technology addresses the reduction in the output power of semiconductor technologies at higher powers, alongside the increased propagation loss, thanks to a combination of concentrating the emitted power into a focused beam and enhancing the sensitivity at the receiver.
“This array-gain benefit lowers the power-per-element that is required,” explained Cahoon. “In addition, the number of elements that can fit within an given aperture size increases with frequency squared, so that the end result is that even when we move to sub-terahertz frequencies, we can maintain the same link budget as, say, a 5G millimetre-wave phased array, using a lower power output per element, and having a smaller physical array aperture, but yet within that array having many, many more elements.”
As fT and the maximum oscillation frequency, fmax, need to be roughly five times that of the carrier frequency used, for 6G these values need to be in excess of 500 GHz. That rule-of-thumb suggests that CMOS and SOI technologies come up short, as they have a performance that plateaus around 450 GHz (see Figure 4). While GaN is also short of that benchmark, peaking at around 420 GHz, SiGe and InP have demonstrated success beyond the high-frequency target.
Figure 4. The maximum oscillation frequency for some semiconductor
technologies is not high enough for them to be candidates for 6G
technology. The rule-of-thumb is that fmax should be at least five times
the value of the carrier frequency.
Cahoon also compared the various technologies for front-end performance, and argued that InP and GaN come out on top. “These material systems have significant advantages over silicon, including a higher breakdown voltage, much higher current and power densities, and a higher electron mobility and saturation velocity.” The upshot of these attributes is a significantly better power amplifier at sub-terahertz frequencies.
However, there are challenges with InP and GaN technologies, warned Cahoon. He pointed out the limited-to-no logic integration, addressed by multi-chip architectures, and the relatively high costs compared with silicon technologies. Cahoon stressed that for all semiconductor technologies, it is imperative to have a comprehensive RF reliability methodology. “This is particularly important at 6G, where we are already challenged for device performance.” Hitting a high level of performance while ensuring reliability will be far from easy. Adding to the challenge with both 5G and 6G is the need to handle complex waveforms with high peaks, a condition that caused devices to move through a range of degradation regimes.
“Thus it’s important to have harder-validated, physics-based reliability degradation models that can be plugged into a reliability simulator that can do lifetime calculations based upon this complex waveform.”
Cahoon also reviewed D-band performance of PAs produced with various technologies. He pointed out that the power-added efficiency of silicon is low, at around 13 percent. InP has the highest figure in this regard, hitting 32 percent, while GaN produces the highest output power. For the low-noise amplifier, it’s a similar story, with InP offering the lowest noise figure.
As well as PAs and low-noise amplifiers, phased-arrays are needed for 5G and 6G networks. Cohen introduced this topic by describing a typical 5G 28 GHz state-of-the-art array, made by Analog Devices. Formed with a 45 nm silicon-on-insulator process, this 256-element antenna-on-board phase array had chips on one side of the PCB and the antennae elements on the other. With each chip dissipating about 5 W in this 50 mm by 50 mm array, there is a dissipated power density of around 10 W cm-2. To extract the heat, there is a large heat sink in thermal contact with the chips.
To progress to 140 GHz arrays, suitable for 6G, the dissipated power density threatens to spike, but must be maintained to 10-20 W cm-2. This plays into the hands of compound semiconductor devices. “We can see the traction of the higher efficiency of indium phosphide for reducing total array power, but this comes with the challenges of the lack of integration capability and the high cost of indium phosphide today.” So it might be that SiGe devices are adopted, driven by current efforts to improve its high-frequency capability. With 6G still some way off, it’s hard to tell at this stage what role GaN will play there. But for millimetre-wave 5G, there’s a great opportunity, supported by efforts to deepen the understanding of impediments to reliability and how to address them. Success on this theme promises to be one of the highlights of the IRPS meeting for several years to come.