
Supporting a surge in UVC optoelectronics
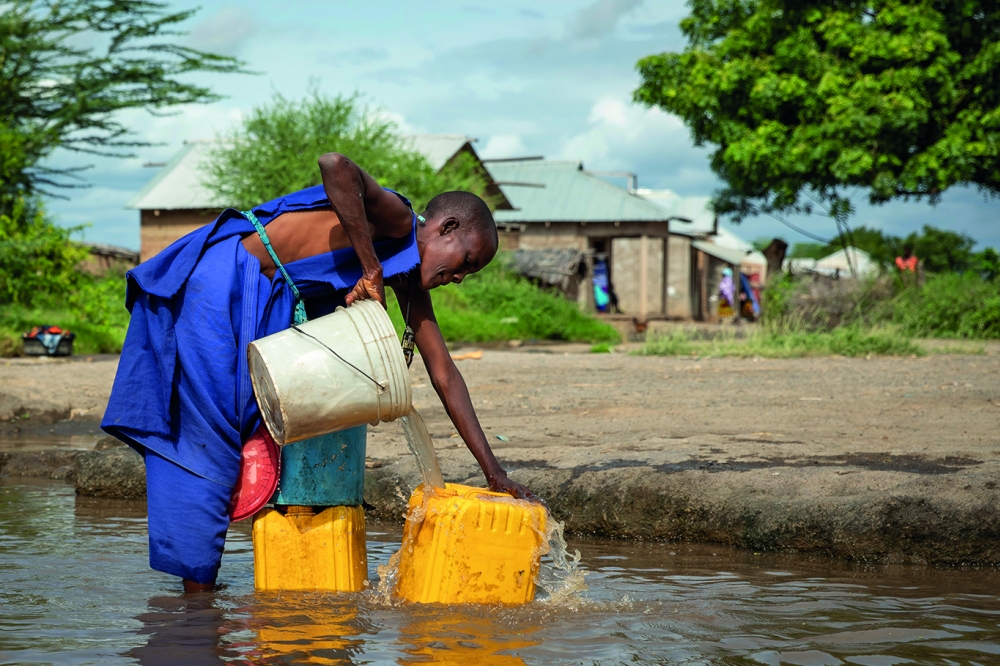
Defect control during the growth of AlGaN layers on AlN substrates
ensures record-breaking performances for UVC LEDs, lasers and avalanche
photodiodes.
BY PRAMOD REDDY, RONNY KIRSTE, RAMON COLLAZO AND ZLATKO SITAR FROM ADROIT MATERIALS.
Our views on what is a basic human right will differ from person to person. But the vast majority of us will agree that all of humanity should have access to clean drinking water. This view is supported by the United Nations General Assembly, which has explicitly recognised the right to water and sanitation, acknowledging that both are essential for realising all human rights. Helping to ensure that this will happen is a resolution from 2010: it calls upon countries and international organisations to support efforts to provide safe, clean, accessible and affordable drinking water for all, by providing financial resources, helping with capacity-building and aiding the development of relevant technology.
One leading option for ensuring that water is safe to drink is to treat it with UV light, as this destroys the DNA of microorganisms. While this is also possible with chemical treatments, UV light offers the safest, most reliable and sustainable way to free water from microbial contamination.
Additional applications involving of the use of UV light for the benefit of mankind include the sterilisation of air and surfaces. The pandemic has piqued interest in this, with sales of UV light sources increasing to combat the virus.
The incumbent technology for providing UV light is the mercury-based lamp. However, it’s far from ideal, as it is bulky, fragile, and employs toxic material. Offering far more promise is a chip-based technology, the LED, which can operate in the UVC.
Figure 1. (left) Potential applications for UVA (360–320 nm), UVB (315–280 nm), and UVC (280–200 nm) laser diodes. (right) Bandgap emission of GaN and AlN, illustrating that the ternary semiconductor AlGaN can cover the UVA, UVB and UVC wavelength range.
Semiconductor-based devices that operate in the UVC are also promising candidates for the detection of light. Again, it’s the story of replacing an incumbent technology shrouded in glass – in this instance a photomultiplier tube – with a chip-based alternative, this time the avalanche photodiode (APD). The latter is compact, lightweight, runs off a low voltage, ensures long-term operation with highest reliability, and has excellent detection characteristics: high sensitivity; substantial gain; and excellent solar spectrum rejection, implying that it is hardly affected by stray light.
Thanks to all these attributes, avalanche photodiodes operating in the UVC have the potential to be deployed in many applications. As well as being used in atomic clocks for autonomous and deep-space operation, due to the development of mid- and far-UV detector arrays, these devices could serve in astronomy, space observation, quantum sensing, and other fields with UV photography.
LEDs and photodetectors operating in the UVC, as well as lasers that emit in this spectral range, share the same material system. All these devices are made from the ternary alloy AlGaN, which has a bandgap that varies from 3.4 eV to 6.03 eV – equating to 365 nm to 205 nm (see Figure 1, which details the wide variety of applications where this alloy, which has immense potential, could find deployment).
Figure 2. X-ray diffraction 2θ-ωscan of an AlN homoepitaxial layer in
comparison with the substrate (left) and high-resolution transmission
electron microscopy of an homoepitaxial AlN film showing perfect lattice
continuation across the interface (marked with arrows) (right).
The challenges …
Various factors within the device layers are impeding the realisation of AlGaN-based optoelectronics. Developers of these devices are having to contend with challenges within the layers, related to doping and non-radiative recombination, and difficulties at the material surface that lead to the likes of poor ohmic contacts and ineffective passivation.
The primary source of all these impediments arises from crystal defects. They include: extended defects, such as dislocations; and point defects, including impurities, vacancies, and their complexes. When AlGaN-based heterostructures are grown on substrates such as sapphire and SiC, the large degree of lattice mismatch with the epilayers results in high threading dislocation densities.
The imperfections in the material of these UVC LEDs leads to low efficiencies and a compromised output power, preventing them from being suitable for sterilisation. These deficiencies also account for the lack of demonstration of a laser with AlGaN-based quantum wells that emits at wavelengths below 300 nm, and the absence of commercial APDs and other detectors based on AlGaN. While several groups have reported APDs with AlxGa1-xN layers with a high aluminium content (x > 0.4), grown on sapphire substrates, these detectors are impaired by a trade-off between device area and signal gain. Primarily, performance is limited by high screw dislocation densities, linked to both the premature breakdown of the diodes under reverse bias and the high noise levels associated with leakage currents. Note that there is yet to be a demonstration of Geiger-mode operation with AlGaN-based photodiodes.
Figure 3. (left) High-resolution transmission electron microscopy
micrograph for AlGaN on AlN for a nominal 80 percent aluminium content;
no dislocations were observed at the heteroepitaxial interface or in the
AlGaN. (middle) Atomic force microscopy image of Al0.7Ga0.3N grown on
vicinal (0001)-oriented AlN. The surface consists of desired bilayer
steps with a root-mean-square roughness of less than 50 pm. (right)
Scanning tunnelling electron microscopy image of an
Al0.55Ga0.45N/Al0.65Ga0.35N multi-quantum well structure with an
electron-blocking layer. All wells and barriers are well defined and of
homogenous composition.
Another major challenge facing those that are keen to develop UVC optoelectronics with AlGaN is that at higher aluminium contents the activation energy of the magnesium-acceptor increases. An unwanted consequence is the promotion of compensating point defects, such as nitrogen vacancies. The upshot of these trends is that it’s challenging to realise p-type doping with aluminium-rich AlGaN and produce an associated p-type conductivity. Resistivities are typically more than 50 Ω cm, hindering carrier injection and introducing a significant power loss that’s a drag on efficiency. As well as these electrical challenges, adding magnesium induces absorption losses in the waveguide, preventing laser operation.
To address poor ohmic contacts associated with aluminium-rich AlGaN, many device designers employ a magnesium-doped GaN hole-injection layer. Keeping this layer thin enables ohmic contacts and efficient carrier injection, but at the expense of absorption in the desired UV spectrum. The resulting heavy absorption losses impact emitters and detectors.
Fortunately, these issues don’t plague n-type doping of AlGaN, which has been established for aluminium-contents of up to 80 percent. However, device developers still face a point-defect problem in AlGaN. Quashing peak conductivity is a high doping limit that results in self-compensation through vacancies and complexes; and there’s also a low doping limit, due to impurities such as carbon, that introduces recombination centres in quantum wells and leakage in multiplication regions. Due to these issues, point defect control is a pre-requisite for high-performance UVC optoelectronics.
…and the solution
At Adroit Materials of Cary, NC, we are working with partners to support the production of electronic and optoelectronic devices offering expertise in the growth, characterisation and delivery of state-of-the-art epitaxial AlGaN-based structures and templates. Key to the growth of high-quality material is our defect-control technology, which includes extended defect control via homoepitaxy on AlN and GaN single-crystal substrates.
The foundation for our work is the high-quality AlN boule, grown by physical vapor transport and licensed and commercialised by several companies. Substrates cut from these boules have an average threading dislocation density below 103 cm-2 – that’s a million-fold improvement on threading dislocation densities of more than 109 cm-2, seen in AlN/GaN grown on sapphire.
According to X-ray diffraction studies and high-resolution transmission electron microscopy, when we grow thin films of AlN on AlN single-crystal substrates no new defects form at the interface and there is perfect lattice continuity (see Figure 2). Thanks to this foundation, when we undertake heteroepitaxy of AlGaN on AlN substrates with pseudomorphic growth, our epistructures are free from threading dislocations and have atomically smooth surfaces. These merits enable near ideal interfaces and multi-quantum well structures with exceptional quality (see Figure 3). Drawing on this growth technology, we can use single-crystal substrates as the foundation for nearly extended defect-free AlGaN films constituting the various active layers of lasers, LEDs and APDs.
It’s important to note that the growth of AlGaN on substrates made from GaN results in tensile strain and cracking. So, to realise high-quality thin films on that alternative foundation, there’s a need for facet-controlled epitaxial layer overgrowth, a more complex technique. We have developed point defect control frameworks, such as chemical potential control and defect quasi Fermi level control. These technologies enable us to pursue a targeted reduction of impurities in AlGaN layers of devices. In turn, we have been able to push the doping limits for AlGaN and enable improved electrical conductivities and carrier transport, alongside reductions to thermal and non-radiative recombination losses.
Figure 4. (a) Conductivity of silicon-doped Al0.7Ga0.3N on sapphire and
AlN. (b) The non-radiative coefficient (A) is reduced by several orders
of magnitude in samples grown on AlN due to a low dislocation density,
along with point defect control, resulting in (inset) photoluminescence
with an internal quantum efficiency of more than 90 percent. (c) A
comparison of APD performance with AlGaN on AlN substrates with those
obtained on AlGaN grown on sapphire and (inset) a packaged APD on AlN.
(d) Record low UVC laser threshold of optically pumped lasers achieved
on AlN substrates and a micrograph of a fabricated laser structure.
Every aspect of device performance benefits from our extended and point defect control. By mitigating self-compensation in silicon doped n-AlGaN, these layers can realise conductivities as high as more than 150 S cm-1. Non-radiative recombination rates are exceptional, with a three-orders of magnitude improvement ensuring a record-breaking internal quantum efficiency of more than 90 percent, thanks to the use of our defect control frameworks. Drawing on these virtues, we have demonstrated AlGaN multi-quantum well laser structures with record low lasing thresholds of less than 10 kW cm-2 (note that the first electrically injected UVC laser diode and the best optically pumped laser diodes had single crystalline substrates for their foundation). Finally, we have demonstrated a nearly two orders of magnitude improvement in APD performance, in terms of the product of gain and efficiency, over a wide range of detection areas. This triumph has resulted in technological viability of solar-blind AlGaN APDs.
It is only in the last ten years that major progress has been made in the field of AlGaN UVC optoelectronics. Compared with other optoelectronic devices, that’s not that long. Consider, for example, the GaAs laser diode, which took decades to mature before widespread commercialisation. While recent progress may not be obvious, tremendous advances have been made in this field, creating a very positive outlook.
Further reading
R. Kirste et al. “Status of the growth and fabrication of AlGaN-based UV laser diodes for near and mid-UV wavelength” J. Mater. Res. 36 4638 (2021)
P. Reddy et al. “Point defect reduction in wide bandgap semiconductors by defect quasi Fermi level control” J. Appl. Phys. 120 185704 (2016)
P. Reddy et al. “Point defect reduction in MOCVD (Al)GaN by chemical potential control and a comprehensive model of C incorporation in GaN” J. Appl. Phys. 122 245702 (2017)
K. Wang et al. “The role of Ga supersaturation on facet formation in the epitaxial lateral overgrowth of GaN” Appl. Phys. Lett. 120 032104 (2022)
Z. Zhang et al. “A 271.8 nm deep-ultraviolet laser diode for room temperature operation” Appl. Phys. Express 12 124003 (2019)