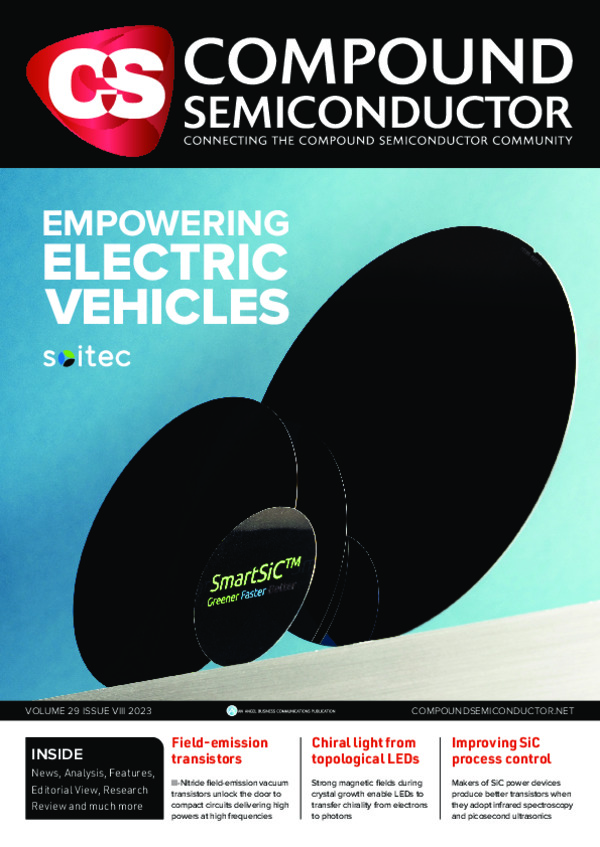
Realising chiral emission with topological LEDs
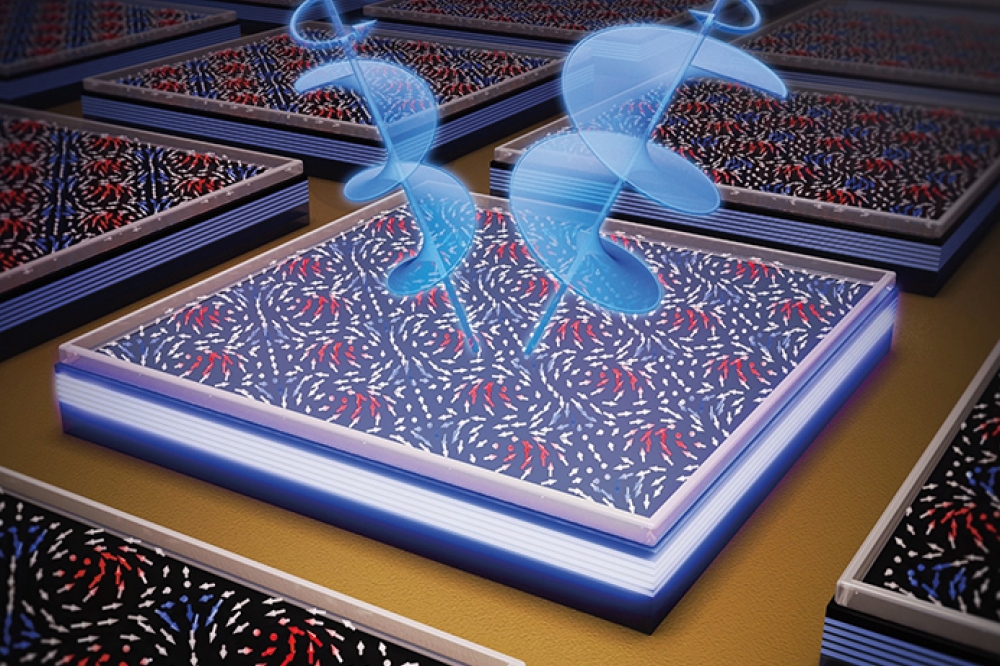
Applying strong magnetic fields during crystal growth enables the
fabrication of LEDs that transfer chirality from electrons to photons.
BY JUNYONG KANG FROM XIAMEN UNIVERSITY
The revolution in the sharing of information is underpinned by the highly efficient processing, storage and transmission of data. For the latter, information is sent and received over optical networks, with data encoded through effective modulation of the amplitude or phase of the transmitted light. However, despite the ubiquity of this approach, it is not without weakness. One major drawback is that external perturbations and attenuations compromise the quality of this form of optical communication. So significant is this issue that the technology of today will fail to meet the challenges associated with tomorrow’s wide-band, high-speed data transmission.
Offering a solution to this important problem is chirality, an intrinsic feature of the photon. By encoding photons with different chirality, namely either the left-handed or the right-handed form, networks will be able to efficiently transfer binary information while offering a much stronger resistance to background noise. That’s not the only merit, however: encoding light with phase and chirality will also enable highly stable quantum key distribution, crucial to secret communication.
The chirality of a photon is closely related to the spin of an electron. To ensure simplicity and elegance, rather than using polarised optical elements to realise chirality, existing chiral photon sources employ spin-polarised materials. Such structures enable the transfer of electron spin to photon chirality via spin injection. However, chiral selectivity is far from ideal, due to constraints in spin polarisation and spin injection efficiency. Additional impediments to producing high-performance, practical systems are the need to use external magnetic fields or low temperatures to suppress electromagnetic or thermal perturbations. Substantial strides have to be made in stability and polarisation, in order to develop high-performance chiral photon sources.
Figure 1. Large-scale meron lattices produced by high magnetic field MBE and observed by magnetic force microscopy.
Turning to topology
A very promising way forward involves the use of a relatively new class of materials. For this particular family, characteristics depend not only on what the material is made from, but how it is arranged. Due to this rather striking behaviour, such materials are known as topological structures, named after a branch of mathematics known as topology.
Researchers discovered the first topological structures, which were insulators, as far back as 2007. In such materials, electrons zip along edges or surfaces in a manner that does not change under deformation of the material. Over the intervening years, the range of topological materials has expended to include photonic topological insulators – structures with photons zipping around the edges and surfaces – and topological superconductors and semimetals.
Within this growing family are those that involve the spin of the electron. Within this subset are skyrmions and merons – they feature vortex-like spin textures in magnetic thin films that exist out-of-plane and in-plane, respectively. Due to their unique topological protection features, skyrmions and merons have a higher stability than conventional electronic materials.
Our team at Xiamen University is trying to seize on this asset, which promises to address the stability bottleneck in polarised materials. Breaking new ground, we are pioneering the introduction of topological spin structures for chiral photon sources.
Figure 2. (a) Overfocused Lorentz transmission electron microscopy images of meron pairs with alternated dark and white contrast, measured under zero magnetic field and at 300 K. Scale bar, 200 nm. (b) Schematic of the meron pairs where in-plane spin component distributions were deduced from the selected domain region at 300 K using the transport-of-intensity equation. The inset shows the colour wheel for the direction of in-plane magnetisation.
We are not the first to produce a topological spin structure. There are already a handful of reports of experimental success by other groups. However, device applications are hindered by a limited lattice scale, as well as strict temperature or magnetic field requirements. What’s needed to realise the practical application of topological spin structures in chiral photon sources is the fabrication of large-scale ordered topological lattices that exist at room temperature and without a magnetic field – with the latter of these requirements needing to be solved first.
Success on this front needs the nucleation of topological order in magnetic systems. This can be accomplished with a strong Dzyaloshinsky Moriya interaction (DMI), which is an asymmetric exchange interaction originating from strong spin-orbit coupling. The DMI is associated with the orientation of d-orbitals in typical metallic magnets. Unfortunately, manipulating the orbital orientation is challenging, with no solution forthcoming for many years.
Offering a breakthrough is our latest work, which draws on our experience in growing magnetic crystals under high magnetic fields. Our progress has led us to propose a new principle: orbital-regulated topological protection. Based on theoretical simulations, we have established that a strong magnetic field during crystal growth can enhance and freeze orbital coupling, thereby improving crystalline and spin ordering and ultimately inducing a strong DMI. This finding promises to provide the foundation for the nucleation of large-scale topological lattices that are stable at room temperature and under zero external field – essential conditions for practical devices.
Figure 3. Trajectory of electrons injecting through a meron pair. The purple balls with the letter ‘e’ are electrons. Purple arrows illustrate the directions of electron spin after injection.
Motivated by this tantalising possibility, we have developed high-magnetic field-assisted MBE equipment, which has been subsequently patented in China and the United States. Following systematic selection of materials, we have settled on a Pd/Fe/MgO trilayer structure, which offers broken spatial inversion symmetry when grown on a GaN substrate. During the deposition of iron, we apply an in-situ magnetic field of up to 9 T. According to our comprehensive structural and magnetic characterisation, samples grown under a 9 T magnetic field exhibit superior crystallinity and a higher magnetisation. Measurements reveal a strongly DMI (−0.4266 mJ m-2) and a dominant in-plane magnetic anisotropy, which are enhanced compared to those grown without any magnetic field.
We have been intrigued by finding paired circular magnetic domains with opposite polarities. They were uncovered by magnetic force microscopy (see Figure 1) and Lorentz transmission electron microscopy (see Figure 2). They provide confirmation that we constructed topological meron-antimeron pairs, and also reveal a Bloch-type spin texture with left-handed spin chirality in meron lattices, consistent with the negative value for the DMI strength. These meron-antimeron pairs exhibit long-range ordering over the 6 mm by 6 mm wafer, forming large-scale meron lattices.
It is worth noting that were observed these meron lattices at room temperature and without a magnetic field. Even after 530 days, our meron lattices exist stably. These findings demonstrate that topological protection can be introduced into the magnetic thin films through orbital-regulated growth.
Figure 4. The topology-induced spin
LED structure and the principle of chirality transfer from injected
electrons to emitted photons in the electroluminescence measurements.
Transferring chirality
One obvious, crucial question is whether the chirality in meron lattices can be transferred to light, to form a chiral photon source. Often this is not considered, because topological spin structures tend to be viewed as information carriers for future information devices, and their opportunities in optoelectronics are overlooked. Current research is focused on manipulating topological spin structures using light or spin current – there are, for example, efforts directed at the likes of race-track memories and skyrmion logic gates – while the manipulation of electrons and photons by topological spin structures is not on the agenda.
Helping to reset the balance, we have analysed the trajectories of electrons vertically injected into the meron lattice (see Figure 3). We have found that the chiral spins in the meron lattices act on the incident electrons as vortex magnetic fields. Consequently, when electrons pass through these vortices, they are deflected by an additional Lorentz force without an external magnetic field. Due to the particularly small core size of the merons, this force is strong enough to dispatch electrons to regions with different spin polarisations.
It is possible to calculate the degree of spin polarisation produced by a meron-antimeron. In the meron area, the force converges the electrons into the core region, causing them to be polarised along the core spin. Meanwhile, in the antimeron area, the force ensures divergence, driving half of the injected electrons to the adjacent meron area. Due to both of these activities, the number of output electrons in these two areas differs, inducing a spin polarisation of up to 50 percent in the output current – and revealing the potential for chirality modulation for electrons.
Following identification of the modulation effect in electron spin, we have integrated meron lattices in nitride-based LEDs, using a Pd/Fe/MgO trilayer as the spin injector. With this novel structure, the injection of spin-up and spin-down electrons produces electroluminescence with right (σ+)-circular and left (σ−)-circular polarisation, respectively – as expected from the selection rules for electronic transitions (see Figure 4).
The degree of chirality of the emission is governed by the strength of the magnetic field during MBE growth. For the 0 T sample, circular polarisation is just 0.9 percent, so essentially neglectable, while the 9T variant produces a record-high circular polarisation of 22.5 percent (see Figure 5).
Figure 5. (a) Circularly polarised
electroluminescence spectra of the topology-induced spin LED (T-LED)
grown under 9 T magnetic fields. (b) Comparison between the T-LED and
nitride-based spin LEDs, using results reported in the literature. The
T-LED has a surface-emitting geometry. This ensures a substantially
higher luminous efficiency than that for an edge-emitting geometry, due
to an anisotropic carrier transition.
We attribute the tremendous hike in polarisation to the modulation of electron spin from the topological meron lattices that form under the high growth-magnetic-field. Due to this, we describe our device as a topology-induced spin LED. Its high degree of polarisation is direct evidence of effective chirality transfer from topological spin structures to electrons and then photons. Note that this behaviour occurs under practical working conditions for the LED – that’s room-temperature operation under zero magnetic field with an applied current of 1 mA.
Thus, our topology-induced spin LED breaks through the constraints of poor stability and low polarisation, weaknesses impairing existing chiral photon sources.
Key to our breakthrough is our high-magnetic-field MBE growth technology, which manipulates interactions within strong-correlated materials through orbital control. Note that this is a versatile approach that could be employed for controllable growth of other crystals and topological lattices, such as skyrmions and vortices.
As the large-scale topological meron lattices created in our work have room-temperature and zero-field stability, they provide an ideal platform for the frontiers of photonics research. That’s because our on-chip chiral photon source is capable of transferring chirality from topologically protected quasiparticles to fermions with mass and further to massless bosons. It’s a breakthrough that as well as having scientific significance in its own right is a significant step towards the practical applications of topological spin structures.
• The author would like to acknowledge the financial support from the National Natural Science Foundation of China (Grants 61227009, 62022068, and 62274139) and CREST, the Japan Science and Technology Agency (grant no. JPMJCR20T1).