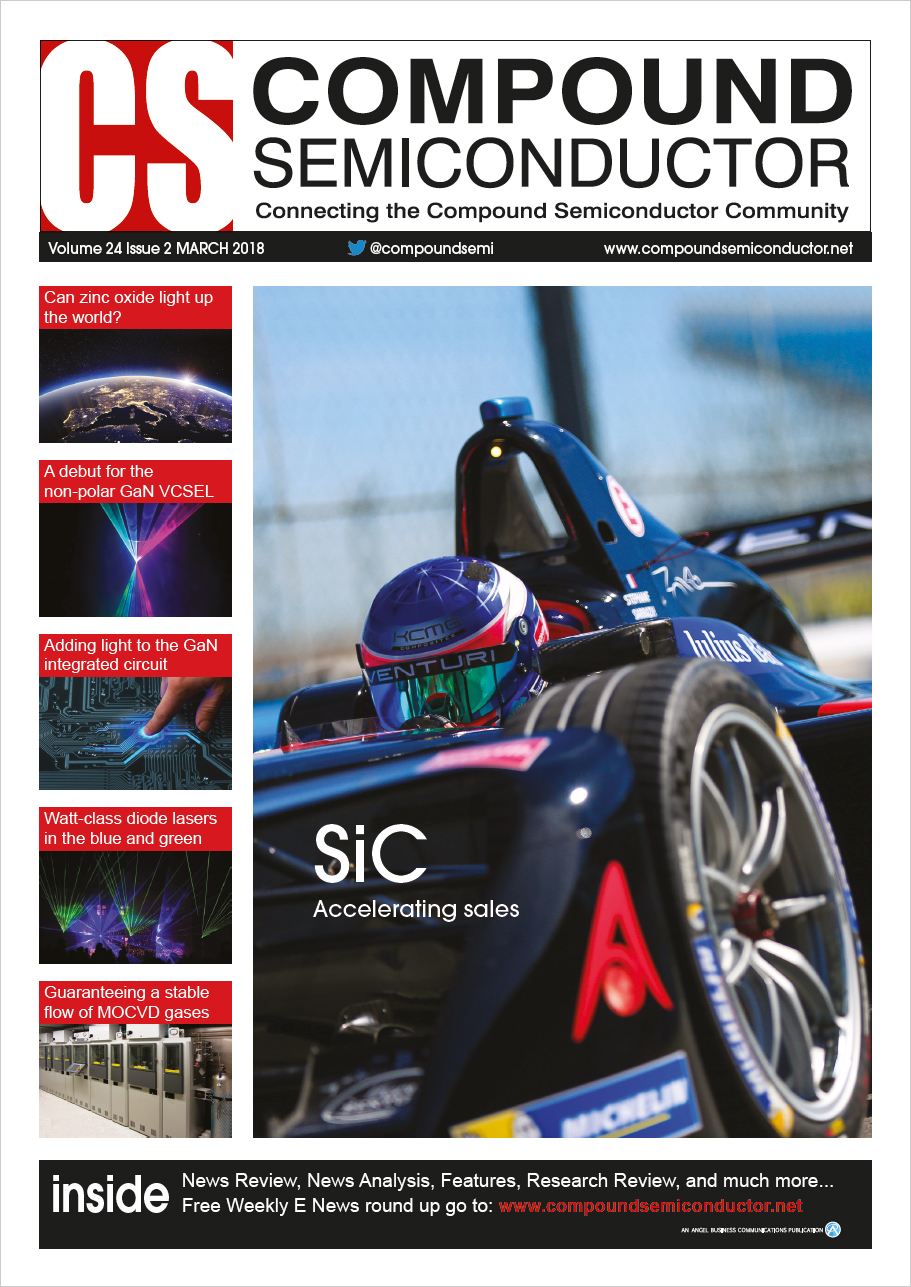
Making monolithic integrated systems with GaN
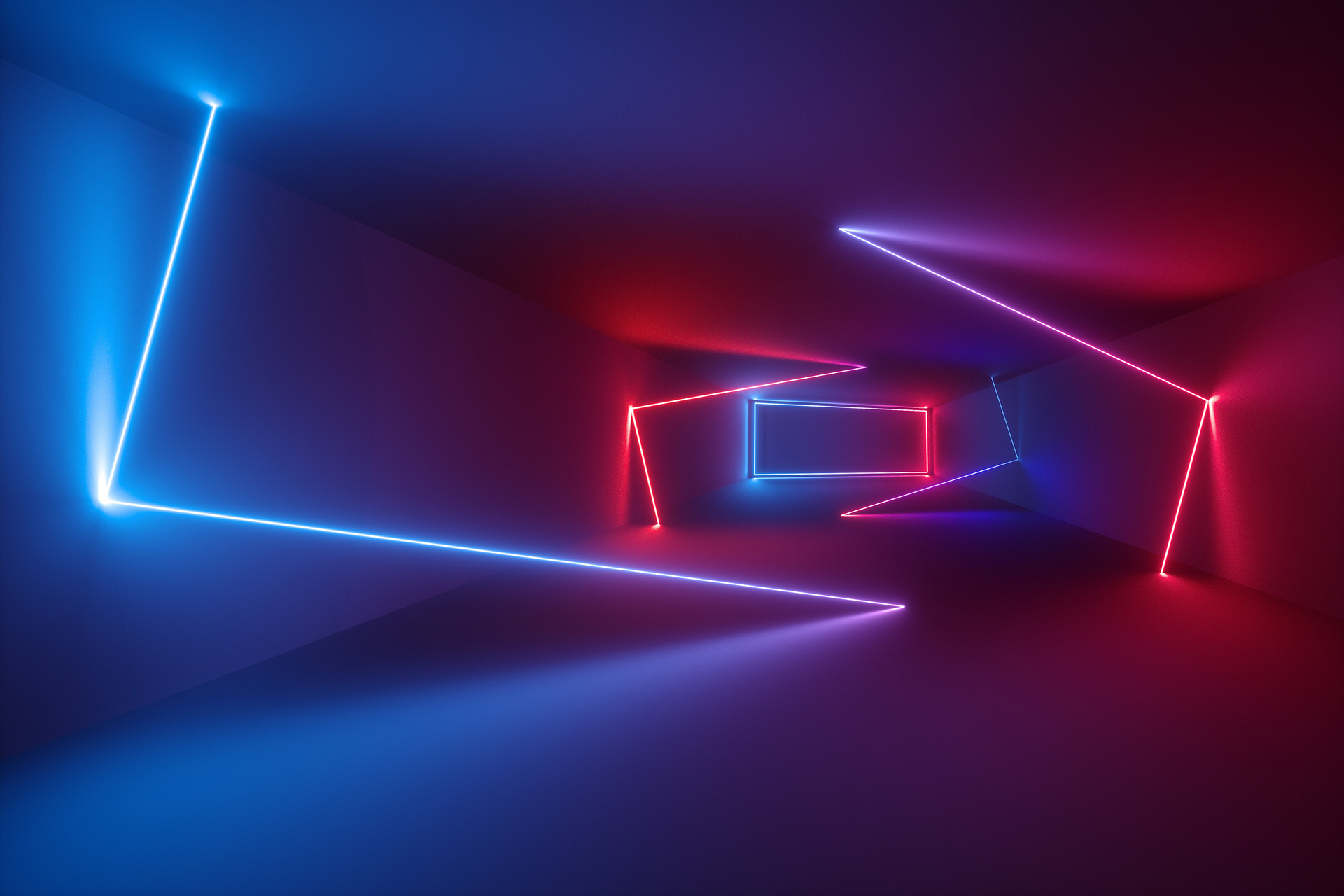
GaN produces great LEDs, lasers and transistors. Just imagine the
possibilities when two or more of these classes of devices are united on
the same chip by Hoi Wai Choi, Wai Yuen Fu, Kwai Hei Li and Yuk Fai
Cheung from the University of Hong Kong
GaN has breathed new life into LEDs. This wide bandgap material has propelled the status of this device from indicator lights to illumination lamps that are pushing incandescent lamps to the brink of existence, while slashing carbon footprints. And the penetration of this device still has further to go, due to rising sales of LEDs that combine GaN with AlGaN, rather than InGaN, the key material for visible emission. This alternative alloy widens the bandgap, enabling the production of devices stretching into the ultraviolet, which can be used to disinfect air, water and surfaces. There is also the cousin of the GaN LED, the GaN laser. It is already being widely used in highdensity optical storage, commonly known as BluRay.
Following in the footsteps of these optoelectronic devices is an electronic industry based on the same family of materials. Their great intrinsic material properties has led the GaN HEMT to be widely touted as the next generation of power electronic device. Further commercial success may follow from other types of GaN device, including microelectromechanical systems and resonators, which are both under development.
In hindsight, it would have been better to develop the broad portfolio of GaN devices along similar lines. But that's not been the case. Instead, devices have often been developed individually, due to the lack of a common structure or platform. Nevertheless, optoelectronics and electronics often go hand-inhand; an LED requires an electronic driver for stable operation, and this driver is built from electronic components. So it is clear that the GaN platform can become even more useful, functional and efficient when multiple components are tightly integrated to form an integrated system, just like that found with silicon ICs (see Figure 1, which illustrates this concept).
Let's begin by considering photonic integrated systems. A promising building block for these is the LED, which combines high quantum efficiencies with fast response times, making it suitable for transmitting signals at high speeds. However, the LED needs to be paired "“ on the same chip "“ with a photodetector capable of detecting the light that it emits. Although the GaN epitaxial layer underneath the active region is capable of making a good photodetector, this would not detect visible light. To address this, a thick InGaN layer may be grown over the LED structure to provide light absorption. However, there is a penalty to pay "“ an increase in the complexity of the process flow.
To avoid this stumbling block, our team at Hong Kong University adopts a different approach, using multiple quantum wells to detect the light that is emitted by this active region. There is much merit in this idea, given luminescence and absorption are complementary optical processes.
With this approach, the detection sensitivity depends on the relative shift between emission and absorption spectra, which is known as the Stokes shift. Despite this limitation, typical InGaN/GaN multiple quantum wells can still have a spectral overlap between 20 nm and 30 nm.
Figure 1. GaN has the potential to make very powerful ICs, by integrating lasers, LEDs and ransistors on a single chip.
As our detectors are positioned adjacent to the LEDs, we can use them to monitor the light output intensity. We have found that the fluctuations and variations in the photocurrent that is generated track those of the light output remarkably well. By using the photocurrent as a feedback signal, we can realise a constant light output intensity, via adjustments in the drive current.
Many applications could benefit from this technology for forming intensity-stabilized emitters. Such sources are needed for optical spectroscopy and fluorescence imaging, and for the calibration of detectors. What's more, intensity stabilisation could ensure stable colour chromaticity in white-light sources formed from red, green and blue LEDs.
Photonic circuits
As LEDs are omni-directional emitters, light-guiding offers the only feasible means of optical transmission. The good news is that GaN, with its high refractive index, is ideal for forming waveguides with air as the surrounding medium. To ensure optimal coupling, waveguides should be physically connected to the emitters and detectors. The top and side facets of the etched waveguides provide excellent optical confinement, thanks to an interface with air. However, there can still be severe leakage through the bottom of the device, which interfaces with the substrate.
Figure 2. The combination of strain and the scaling of the dimensions of the emitter enables blueshifting and red-shifting of the emission wavelength.
Several solutions have been proposed to overcome this problem. If the substrate is made from silicon, a wet-etch undercut process can selectively remove material from underneath the waveguide to form airsuspended waveguides. This approach can draw on the technique that we developed to promote optical confinement in suspended GaN microdisks. Note, however, that this technology cannot be applied to LEDs with a substrate made from sapphire, which is the more common platform for this device. In this case, a selective laser lift-off process can remove the substrate underneath the waveguide.
So, in short, regardless of whether GaN LEDs are formed in silicon or sapphire, there are processes that can generate suspended and even flexible waveguides, enabling optical transmission across multiple planes on the chip.
To see how quickly signals can be transmitted from the emitter to the detector, via the waveguide, we applied a pseudo-random binary sequence signal to our LED and detected the transmitted optical signal with our multi-quantum well photodetector. An oscilloscope, monitoring the photocurrent, produces a widely open eye diagram for signals up to 250 Mbits/s, and determines that the response time of the system is less than a few nanoseconds. Far faster rates beyond GBits/s should be within reach by switching the modulation scheme to one that is more sophisticated, such as OFDM.
In monolithic systems, single-wavelength transmission is to be expected, because the emission wavelengths of the multi-quantum wells are not tuneable. But that doesn't have to be the case. Due to significant lattice and thermal mismatch, the combination of InGaN wells and GaN barriers creates a highly-strained active region, particularly at longer emission wavelengths, and this offers a viable way of wavelength tuning.
Efforts are aided by dimensional downsizing, which can blue-shift emission wavelengths, due to strain relaxation. Reducing dimensions below 100 nm blueshifts emission by as much as 80 nm, turning green emitters into blue ones, and enabling controllable, multi-wavelength emission from a single wafer. It is also possible to go the other way. Introducing strain into the active region by nano-structuring produces a red-shift in emission wavelength. In this case, the wells themselves are not structured; instead it is the nanostructures formed right on top of them. Due to strain relaxation in the overlaying nanostructures, the wells are compressed laterally by inducing a tensile strain vertically.
Using this approach, we have recorded spectral redshifts of more than 15 nm, propelling the emission wavelength from 572 nm in the as-grown structure to 589 nm. Given the challenge of realising red-light emission in active regions formed from InGaN wells and GaN barriers, our approach offers much promise as an alternative route for producing red-light InGaN LEDs, especially when such LEDs are to be monolithicallyintegrated with LEDs of other wavelengths.
Obviously, we didn't develop wavelength tuning by strain manipulation for multiple wavelength monolithic sources. Instead, the motivation for developing this technology was the creation of full-colour displays and monolithic white-light emitters, an attractive alternative to sources that include phosphors.
During this effort, we found that structuring the active region of an LED into an array of multi-dimensioned structures, with sizes ranging from tens of nanometres to several microns, can produce a spectrum that is broad enough to yield white light. The extent of strain relaxation and induction governs the wavelength tuning range. For example, an array of nanostructures and microstructures with dimensions ranging from 50 nm to 5 μm is capable of providing a spectral width of 148 nm and a colour rendering index of 61 (see Figure 2).
This technology can also be used to form full-colour monolithic displays from a combination of wavelength adjusted pixels. The starting point is an LED wafer with green-emitting quantum wells. Blue and red pixels are then formed by introducing strain-relaxed and strain-induced elements respectively (see Figure 3). Note that the creation of red, green and blue pixels on the same chip is a very attractive approach to realising full-colour LED displays, which are currently constructed using pick-and-place solutions "“ they face several limitations, including pixel dimensions and pitches, yields and costs.
Adding electronics
So far, we have discussed the monolithic integration of optoelectronic and photonic components. Even greater rewards could come from the integration of electronic components, as this would greatly enhance the functionalities of the integrated systems.
Some success has already been realised. Kei May Lau's group at Hong Kong University of Science and Technology has reported extensively on the integration of GaN LEDs and HEMTs; and we have combined optoelectronic devices with MOSFETs, a form of transistor that is easier to introduce in the manufacturing process.
Initially, we developed Schottky-barrier MOSFETs to overcome potential issues associated with the shrinking of silicon devices. However, it turns out that those concerns can be resolved - at least for now. Nevertheless, such devices, sporting metallic sources and drains, remain attractive for GaN materials, because they eliminate the need for source and drain doping. Instead, the roles of the p-n junctions are fulfilled by metal-semiconductor Schottky junctions. This approach has great versatility, as both p-channel and n-channel transistors can be formed on the n-GaN and p-GaN layers of the LED wafer. ~
Figure 3. Full-colour displays could be created by taking a wafer designed for green LEDs and using strain and dimensional constraints to introduce structures with red- and blue emitting regions.
The normally-off transistors that we produce, which have high switching speeds, are an ideal building block for the integration of digital circuits onto the integrated system. For instance, placing these transistors (and capacitors) adjacent to the pixels in a monolithic display enables active-matrix addressing without relying on off-chip components. The upshot is a better-performing, more robust system.
Our work showcases the promise of monolithic integration of a diverse range of optoelectronic, photonic and electronic devices and components onto a typical GaN LED wafer. The list of devices we have developed is certainly not exhaustive, however. For instance, whispering-gallery mode microdisks can be integrated on-chip for various detection or sensing applications through modifications of the resonance conditions, and GaN-based MEMs can be used as actuators via the piezoelectric effect. So further work is needed to fully exploit the capability of this monolithic platform, so that it can deliver significant enhancements to the functionalities and performances of today's systems that are formed from heterogeneous integration.