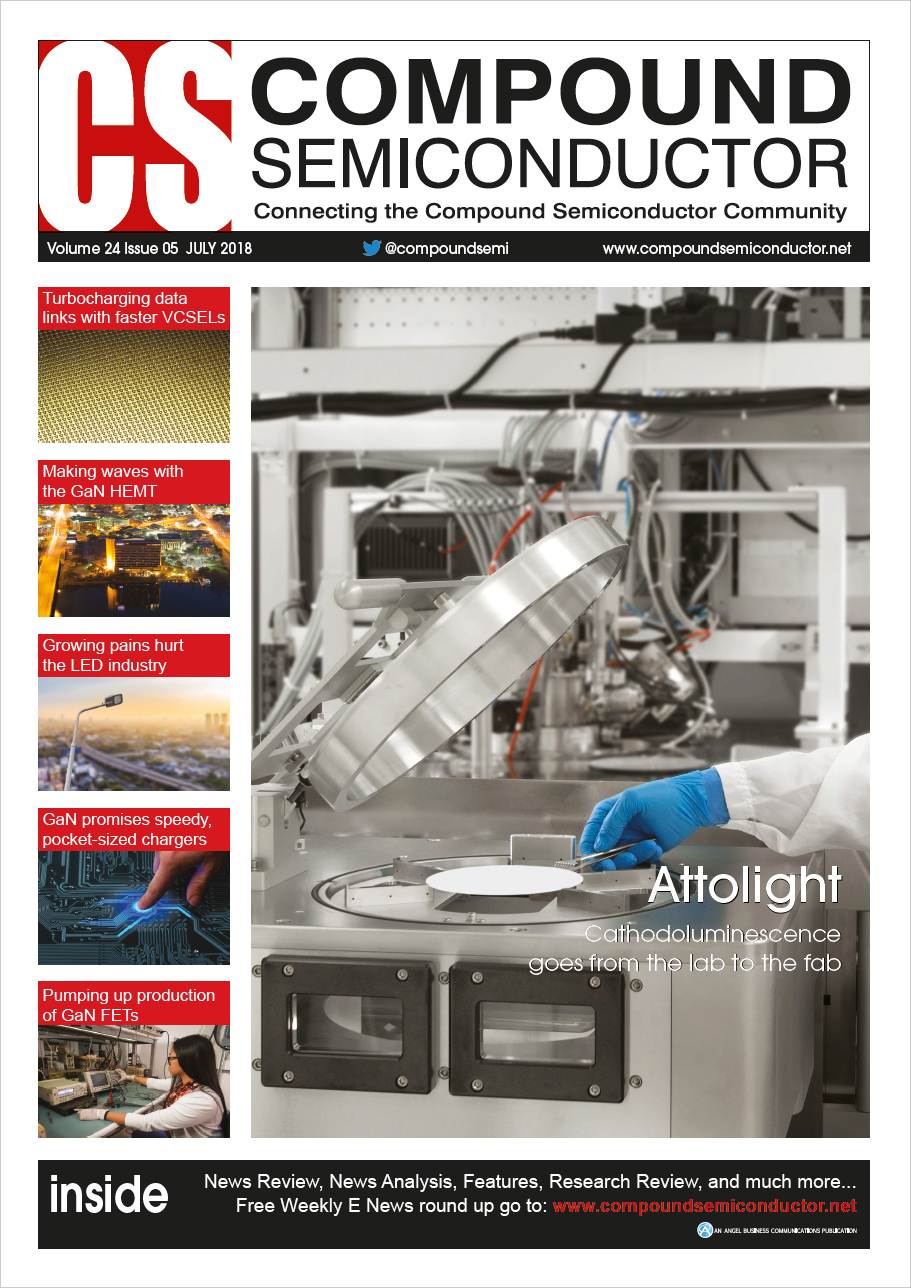
Turbocharging the datalink
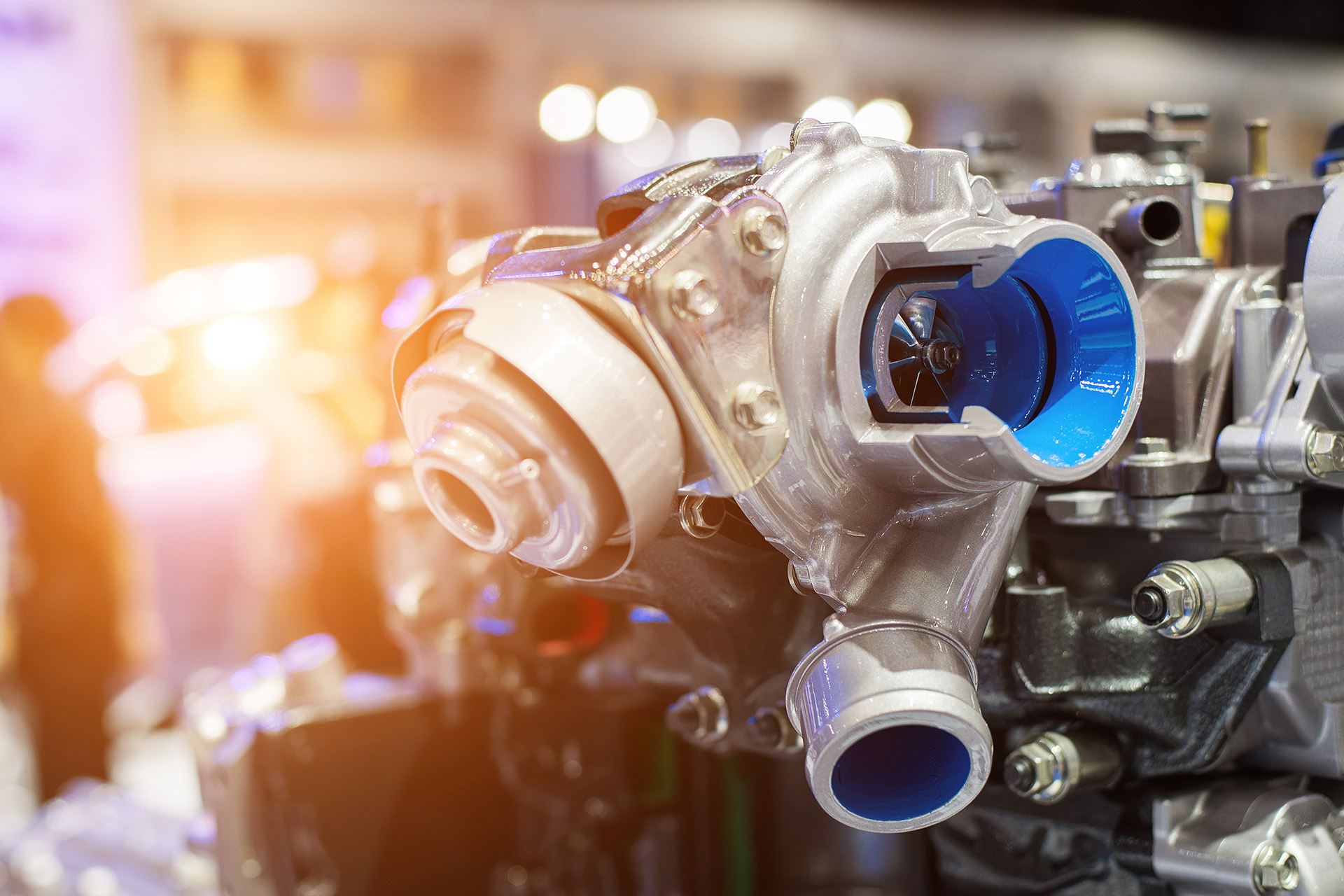
The VCSEL is, without doubt, a highly successful device. Over the last 20 years its shipments have totalled more than a billion, with devices primarily used in data communication and sensing applications.
In the latter market, data coms, the VCSEL is the most common type of laser deployed. First commercialised as a transceiver by Honeywell in 1996, this class of device, predominantly based on AlGaAs and operating at 850 nm, initially offered a line rate of 1 Gbit/s. Today this has increased to 28 Gbit/s, by which time the number of VCSELs in the data centres has climbed to total more than hundreds of millions.
It has taken substantial effort to increase the modulation bandwidth of the VCSEL to enable this speed, while ensuring reliability at elevated operating temperatures. In particular, time has been devoted to optimising the active region of the device and its design.
One secret to realising speeds beyond 10G has been the move from a GaAs quantum well to one made of InGaAs. This ternary introduces strain and increases the differential gain. However, success is not straightforward. Measurements made on about 50 types of VCSELs, differing in architecture and epitaxial growth conditions, reveal that careful design is critical to simultaneously ensuring reliability and speed (see Figure 1). Note that some of these devices were intentionally designed for fast speeds, but poor reliability; and others for slow speeds, but good reliability.
At Finisar of Allen, TX, our first 28G VCSEL product, which we brought to market in 2015, had a reliability that is on par with the preceding 10G VCSEL product. Since its launch in the 28G format, we have made further improvements and tweaks to the reliability of this device, which can be used in Gigabit Ethernet and Fibre Channel technologies. Our refinements have been incorporated in our second-generation product that hit the market in 2017. This successor offers a tenfold increase in time to 1 percent cumulative failure at 80°C, and pushes the expected lifetime to well beyond the ten-year mark (see Figure 2).
In arrays, this improvement in lifetime is particularly welcome. With second-generation devices, a linear array of 1 by 12 VCSELs now has a lifetime of ten years to 1 percent cumulative failure.
We have used several techniques to evaluate the performance of our arrays of multi-mode 28G VCSELs. We have plotted the light-current-voltage characteristics of various arrays (see Figure 3), and undertaken measurements of the small-signal modulation response of these devices (see Figure 4). Results reveal that these 28G products have a typical room-temperature maximum bandwidth of 3dB at around 20 GHz, dropping to about 17 GHz at 95°C. We have also mapped the relaxation oscillation frequency for die across a typical 4-inch production wafer, which provides around 100,000 dies (see Figure 4); and we have recorded typical eye diagrams over a range of temperatures (see Figure 5).
Figure 1. Reliability and speed for a group of different VCSEL designs.
Higher speeds
It is not trivial to produce 28G VCSELs in volume. Therefore, it is a significant challenge to design variants that can provide even higher data rates over a single fibre.
We have had success in this endeavour with a technology known as wavelength-division multiplexing. Since the 1990s, it has been used in the telecom sector, which employs InP-based lasers emitting at 1550 nm.
However, telecom lasers are very different to ours "“ they emit in single mode and transmit over singlemode fibre; whereas the output from 850 nm datacom VCSELs is multimode. Due to this key difference in modal output, at 850 nm the wavelength separation in a multiplexed link needs to be large enough to prevent optical crosstalk and keep mux and de-mux technology cost effective.
Figure 2. Time-to-failure for Finisar's Gen 1 and 2 28G and 10G VCSELs operating continuously at 80°C and worst-case bias current of 8 mA for singlets. Ten years of operation is indicated
Advanced modulation schemes and wavelength division multiplexing offer two routes to speeding 850 nm datalinks to 56 Gbit/s by Petter Westbergh, Deepa Gazula, Gary Landry, Timo Gray, Edward Shaw and Jim Tatum from Finisar
Grid standardization work is ongoing for shortwave wavelength division multiplexing. Currently, the most common scheme is to use four wavelengths: 850 nm, 880 nm, 910 nm, and 940 nm. Today's multimode fibre is optimised for 850 nm "“ and if wavelengths are used that are far from this, modal dispersion will be high and limit the transmission distance of the signal. Due to this, efforts are underway by the like of OFS and Prysmian to develop wideband multi-mode fibre. This technology could create an eight-channel wavelength grid, using additional channels at 970 nm, 1000 nm, 1030 nm, and 1060 nm.
Our 28 G VCSELs for shortwave wavelength-divisionmultiplexing are designed to work over standard multi-mode fibre and deliver a performance that is on par with our 850 nm 28G VCSELs. Thanks to this, our technology can be used to upgrade the transceiver module and increase data rates over existing fibre infrastructure. Options include a ten-fold upgrade to the speed of existing 10G links to 100G, an approach we demonstrated in 2015 at ECOC (see Figure 6).
An alternative approach to increasing speed, which requires only minor changes to existing 28G VCSEL technology, is to change the modulation format for the data that is transmitted. Increasing the number of signaling levels from two to four doubles the amount of information that can be encoded on each symbol. Consequently, the data rate can double while maintaining the same symbol rate, enabling a single channel to support 56 Gbit/s, rather than 28 Gbit/s. This modulation format is called four-level pulsed amplitude modulation.
Figure 3. 28G VCSEL singlet and arrays and typical 28G VCSEL light-currentvoltage plots at 25°C and 95°C.
As all engineers know, there is no such thing as a free lunch. Increasing the number of data levels to detect increases the penalties associated with intersymbol interference and signal noise. The ISI penalty translates to a slightly higher requirement for the VCSEL modulation bandwidth, but this may be met by reducing the size of the aperture (see Figure 7). Reliability then takes a hit, but it is still more than adequate, thanks to the enhanced reliability of our Gen 2 VCSEL material. Time to 1 percent failure for continuous operation of individual elements exceeds ten years.
For transmission with conventional, two-level pulsed amplitude modulation, the relative intensity noise is specified as -131 dB/Hz in both the IEEE and ANSI standards. Moving to multi-level signalling shifts this requirement to -138 dB/Hz, and could limit the yield for the standard 28G VCSEL.
To be able to screen for relative intensity noise, and quickly validate design tweaks that could improve this key figure of merit, we introduced an additional production test system. This enabled relative intensity noise measurements on 100 percent of die on the wafer. Thanks to the insight offered by this test system, we could then identify a path to modifying the design of the VCSEL to improve its relative intensity noise (see Figure 8 for the results of this effort). To showcase our success, we provided a live demonstration of our VCSEL running error-free at 56 Gbit/s at OFC 2017 (see Figure 9).
Even faster data transmission rates can be realised by combining four-level pulsed amplitude modulation with shortwave wavelength-division multiplexing. Working in partnership with engineers at Georgia Institute of Technology, we have used this approach to boost the bit-rate per channel to 107 Gbit/s, and ultimately provide an aggregate bit-rate of more than 400 Gbit/s over a single fibre. This accomplishment, demonstrated at OFC 2017, involved a bit-error-rate for all channels in the range of 10-4 "“ a value that is low enough to enable use with forward-error correction.
Figure 4. Typical 28G VCSEL modulation response at 25°C and 95°C and map showing the variation in relaxation oscillation frequency (ROF) across a 4-inch wafer.
Low latency applications
Our technology for delivering data at 50G over multimode fibre could be used for Ethernet and Infiniband. 50G data transmission appears on both roadmaps, which differ slightly: for Ethernet, fourlevel pulsed amplitude modulation must be used with forward-error correction, while the latter technology is optional with Infiniband.
A major downside of forward-error correction is that it adds latency to the transmission. This is unacceptable in some applications, such as high-performance computing. For four-level pulsed amplitude modulation, error-free low latency transmission is challenging at 50G, due to a significant penalty associated with the signal-to-noise ratio. What's more, four-level pulsed amplitude modulation is less power efficient than the more conventional two-level format. Due to these issues, two-level pulsed amplitude modulation is preferred in low latency applications. This is driving efforts to extend the capability of existing 28G designs.
Figure 5. Eye diagrams for a typical 28G VCSEL at 28 Gbit/s at different temperatures and currents.
To ensure error-free transmission at 56 Gbit/s with twolevel pulsed amplitude modulation while maintaining the link budget, the modulation bandwidth must exceed 30 GHz. Several groups, including Chalmers University of Technology, have demonstrated these bandwidths in laboratory environments with VCSELs that feature an optimised small aperture. However, reducing the aperture size to these dimensions is challenging "“ and doing so in a production line, while ensuring high reliability, is particularly difficult.
Another possibility for squeezing more out of the existing technology is to implement electronic equalization at the transmitter and receiver end. This mitigates the bandwidth limits of the link. Using our VCSELs, and also those from Chalmers University of Technology, IBM has demonstrated this type of link, and produced error-free transmission with two-level pulsed amplitude modulation signalling at bit-rates of 50 Gbit/s and beyond (see Figure 10 for an example). However, there are drawbacks to this approach, such as increases in transceiver complexity and power consumption.
Figure 6. Live demo at ECOC 2015 of a four-channel shortwave wavelengthdivisionmultiplexing VCSEL delivering error-free transmission at 4 x 25Gbit/s over a single 75 m long multi-mode fibre. Note that forward error correction is not used.
With demand for increasing bandwidth showing no sign of abating, a great deal of effort is being devoted to optimising the modulation response of the VCSEL. A few more gigahertz may be squeezed out of this device through incremental improvements in the likes of carrier and optical confinement, differential gain, internal absorption and electrical parasitics. However, it is not clear if this could spur the speed to 30 GHz and beyond. Therefore, efforts are underway on implementing less conventional approaches that promise to bypass the intrinsic speed limit of the VCSEL, set by the finite differential gain and the damping of the laser.
Figure 7. Speed as function of 28G VCSEL aperture size for a group of production wafers.
One potential route to success is to integrate an electro-optic modulator with the VCSEL. This modulator could, in principle, bypass the intrinsic speed limit of the VCSEL, by only being limited by the "˜RC' time constant of the modulator. Vertical integration of the modulator has been attempted by groups that include a team at TU Berlin, while lateral integration has been pursued by researchers at Tokyo Institute of Technology.
Figure 8. Mean diamond box plots of the relative intensity noise (RIN) of different VCSEL designs. Measurements are made on a production RIN tester. The 56G PAM4 (four-level pulsed amplitude modulation) VCSEL, which is indicated, has a part yield that's better than 95 percent for the RIN requirement.
Neither of these options is easy. Challenges include: increased epitaxial complexity; modulating the device without affecting the cavity photon density; and keeping electrical parasitics small, so that high speeds may be realised alongside a sufficiently high output power and extinction ratio.
Figure 9. A live demo of 56 Gbit/s PAM4 (fourlevel pulsed amplitude modulation), given at OFC 2017.
An alternative approach to enhancing the modulation response is to exploit optical feedback through the so-called detuned-loading or photon-photon resonance. We have had success with this, enhancing the modulation bandwidth in edge-emitting lasers beyond 50 GHz. This has led to error-free transmission at more than 50 Gbit/s, using two-level pulsed amplitude modulation (see, for example, our paper from OFC 2016). Optical feedback effects have also been exploited to enhance speed in VCSELs made by groups at Tokyo Institute of Technology, Fuji Xerox, and the University of Illinois at Urbana Champaign. Those VCSELs are producing promising small-signal modulation speeds, but there is a lack of large-signal data transmission results
Figure 10. Eye diagrams for the shortwave wavelength-division-multiplexing VCSEL channels at different bit-rates, along with bathtub curves at 50 Gbit/s, showing error-free transmission using IBM's BiCMOS drivers and equalization.
We are also pursuing alternative approaches to reaching higher VCSEL speeds. Early results are encouraging, with VCSELs producing an optical modulation bandwidth greater than 30 GHz (see Figure 11). We are now evaluating and analysing the commercial viability of these devices.
Figure 11.Normalized modulation response for a candidate VCSEL for 56G operation, using two-level pulsed amplitude modulation.