
Facilitating 5G
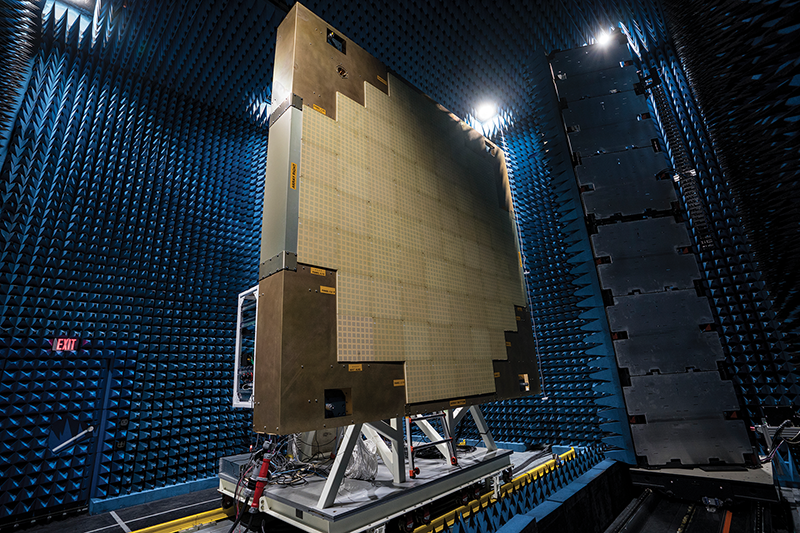
Much has been written about the impending global roll-out of 5G wireless infrastructure. This is expected to have a transformative impact on everything from mobile device connectivity and fixed-wireless services to transportation, industrial and entertainment applications and beyond. By combining data rates that could be ten to a hundred times faster than those of today with the capacity to handle untold data traffic volumes, the global 5G network is anticipated to provide connectivity that is almost instantaneous, alongside a seemingly limitless bandwidth elasticity that will encompass people, autonomous vehicles, IoT devices, industrial systems and civil infrastructure.
Before large scale deployment of 5G can begin, daunting technology and regulatory issues will have to be resolved. These challenges have an upside, however "“ they present a tremendous opportunity for operators to evolve network infrastructure in a sustainable manner that enables continuous improvements in bandwidth, power, management and cost efficiencies, amid an ever-intensifying data deluge. Consequently, the advent of 5G invites a fresh, top-to-bottom look at global communications infrastructure, from RF and optical chips to base station system architectures and network topologies.
It is certainly no coincidence that the efforts at developing 5G are paralleling an evolution in civil radar, which is used for air traffic control and weather surveillance. Efforts at streamlining and improving radar infrastructure are informing commercial 5G technology and deployment strategies. That's not surprising, given that the end goals of these civil and commercial initiatives are similar: the creation of a scalable, cost-effective and highly integrated antenna technology that enables faster, more accurate and more sensitive transmit and receive capabilities. For civil radar, this ensures an expanded field of "˜view' to aircraft and weather systems, while for 5G, it increases subscriber coverage.
In the US, both efforts are supported by government sponsored, cross-agency planning and coordination.
The aim is to consolidate national radar infrastructure into a single multifunction platform, while freeing up valuable radio spectrum for reallocation to commercial 5G wireless services. This programme, known as Spectrum Efficient National Surveillance Radar (SENSR), brings together the Federal Aviation Administration (FAA), the National Oceanic and Atmospheric Administration (NOAA), the Department of Defense (DoD), and the Department of Homeland Security (DHS).
MACOM Scalable Planar Array (SPAR) Tiles
Drawing on civil radar
One of the recent developments in radar antenna has been the introduction of tile-based, planar phased-array radar for air traffic control and weather tracking applications. This breakthrough provides a compelling template for the architecture and assembly of massive MIMO (multiple-input, multiple-output) 5G systems. Drawing on the highly-integrated antenna sub-systems, and volume-scale commercial packaging and manufacturing techniques, will allow those associated with the roll-out of 5G to take little time in building planar arrays that can be flexibly tailored and scaled for deployment across a wide range of environments. And this will be at one-fifth of the cost of that for conventional array architectures, as demonstrated by the first-generation of Scalable Planar Array (SPAR) Tiles targeted for civil applications.
A MACOM 8-inch silicon wafer provides cost efficiencies at scaled volume production
A downside of the current generation of conventional, mechanically-steered air traffic control radars is the slow revisit rates, due to gimbal axis rotation. Planar phased array radars address this weakness with electronic scanning via stationary arrays comprised of hundreds to thousands of transmit and receive elements. What's more, planar arrays are readily equipped to track weather patterns and unmanned aircraft systems, in addition to conventional aircraft. Thanks to this universality, numerous radar functions are integrated into a single, cost-effective multifunction platform.
It is expected that 5G massive-MIMO antennas will adopt a similar architecture to their radar-based cousins. That means that the number of transmit and receive elements will expand well beyond the two to eight antennas found in current MIMO configurations, and extend to anywhere from 64 to hundreds of antennas.
Similarities between planar air-traffic control radar arrays and 5G basestations don't stop at the antenna architecture layer. Both feature a coherent beam forming capability, whereby each transmit and receive element in a massive-MIMO array operates in concert with others. This is highly beneficial, dynamically increasing transmitted power and receiver sensitivity in the direction of subscribers, while mitigating noise, interference and reflections from other sources. In densely populated areas, this approach overcomes bandwidth and capacity limitations, while mitigating multipath problems.
Another virtue of coherent beam forming massive-MIMO is that, by leveraging digital phased arrays, it can outperform traditional fixed-beam massive-MIMO architectures in terms of latency, wide band performance and the use of multiband antennas. The upshot is a superior spectral efficiency, which holds the key to wider coverage areas and a higher quality of service for a greater number of subscribers, at a lower cost of implementation.
Advances in GaN and MMICs
It is not just the evolution in basestation architectures and beam forming techniques that is aiding the deployment of 5G infrastructure. There are also improvements in semiconductor and packaging technologies that are offering compelling advantages over legacy approaches.
For massive MIMO, antenna configurations have to be tightly clustered, creating a need to preserve available PCB space, particularly at higher frequencies. One way to address these constraints is to switch from 5G basestation designs that use discrete ICs and single function MMICs to those that employ multifunction MMICs.
In addition to the space saving advantages provided by multifunction integration, costs can be trimmed by reducing individual die packaging, design complexity, testing and assembly labour. Meanwhile, reductions to the number of interfaces can increase overall mechanical reliability.
With all these factors at play, mainstream adoption of GaN-on-silicon is coming at just the right time. Thanks to scalability to 8-inch and 12-inch wafers, this technology is poised to provide cost efficiencies that are well out of reach for GaN-on-SiC at scaled volume production levels, while delivering performance metrics that can't be achieved with LDMOS.
The superiority over LDMOS is significant "“ power efficiency is more than 70 percent higher, power per unit area is higher by a factor of four to six, and there is scalability to higher frequencies. In parallel, comprehensive testing data has affirmed the capability of GaN-on-silicon devices to conform to stringent reliability requirements, and to replicate and even exceed the RF performance and reliability of rivals made from GaN-on-SiC.
Where GaN-on-silicon clearly has the upper hand over GaN-on-SiC is in its potential to integrate increased functionality at the silicon level. This yields additional space optimization, leading to ultra-compact MMICs; and it enables the homogeneous integration of GaN devices and CMOS-based devices on a single chip. The latter opens the door to multifunction, digitally assisted RF MMICs that incorporate on-chip digital control and calibration, on-chip power distribution networks, and more.
Superior switches
RF switches "“ either in the form of discrete components or front-end modules, where they are integrated with LNAs and controllers "“ will play a pivotal role in 5G wireless basestation performance. There are a wide variety of underlying semiconductor technologies to select from, and designers need to select the best semiconductor junction for the required system function, while keeping an eye on conserving valuable real estate and minimising power loss and associated costs. Insertion loss is a critical performance metric: every tenth of a dB gained in insertion loss performance translates to extended range and expanded subscriber coverage.
For front-end transmit/receive topologies in highpower 5G antennas, extremely low insertion loss can be realised with AlGaAs and heterolithic microwave IC (HMIC)-based switch technologies. Supporting high-frequency operation to enable wider bandwidths, these technologies may help to maximize received sensitivity and antenna coverage while maintaining transmit/receive signal integrity. In addition, by lowering power loss, these technologies may lead to less compensation further down the RF signal chain.
To give an example of these benefits, a discrete heterojunction AlGaAs p-i-n diode switch can halve the high-frequency insertion loss compared to other switches in its class. This advantage translates to a more reliable system performance at higher frequency bands.
Optical opportunities
To handle the massive increases in network data traffic that lie ahead, emerging 5G network architectures are expected to rely more heavily on high-speed optical interconnects from the network backbone through to baseband processing, and on to the antenna. This architecture, which we refer to as fibre-to-the-antenna, reduces copper cabling as new fibre deployments push deeper into the network.
Wireless fronthaul is a prime example of how innovations in optical semiconductor and packaging technologies "“ at optimal cost structures "“ have the potential to transform today's wireless network topologies, which could extend from remote radio units to baseband units.
We anticipate new 100 Gbit/s, single-wavelength PAM-4 DSPs and advanced silicon photonics technologies to provide high-performance, cost effective 100G optical interconnects for centralized/cloud radio access network architectures. This will greatly simplify the design and maintenance of remote radio units, with processing intelligence moving to a centrally managed, maintained, and cooled baseband unit, which services a multitude of remote radio units over long distances.
Previously, the common public radio interface protocol enabled a streamlining of centralized/cloud radio access network architectures, but data delivery speeds fell short of the mark. Bridging this gap is 100G connectivity in cloud data centres, a move that has resulted in a reduction in 100G cost structures. Wireless operators are now well positioned to exploit the speed and cost efficiencies of 100G interconnects for wireless fronthaul in preparation for the 5G data deluge, while enabling a flexible centralized/cloud radio access network architecture designed for seamless scalability into the future.
The parallel advances in RF and optical semiconductor technologies, basestation system architectures and network topologies are converging to propel the wireless networking industry toward the realization of more elegant, integrated and cost effective wireless infrastructure on the path to 5G connectivity. Drawing on innovative technologies honed in the civil and commercial technology domains, system designers and network architects are well equipped to realise higher levels of functionality, performance and device integration for 5G network infrastructure while streamlining data flows throughout the network, from core to antenna.