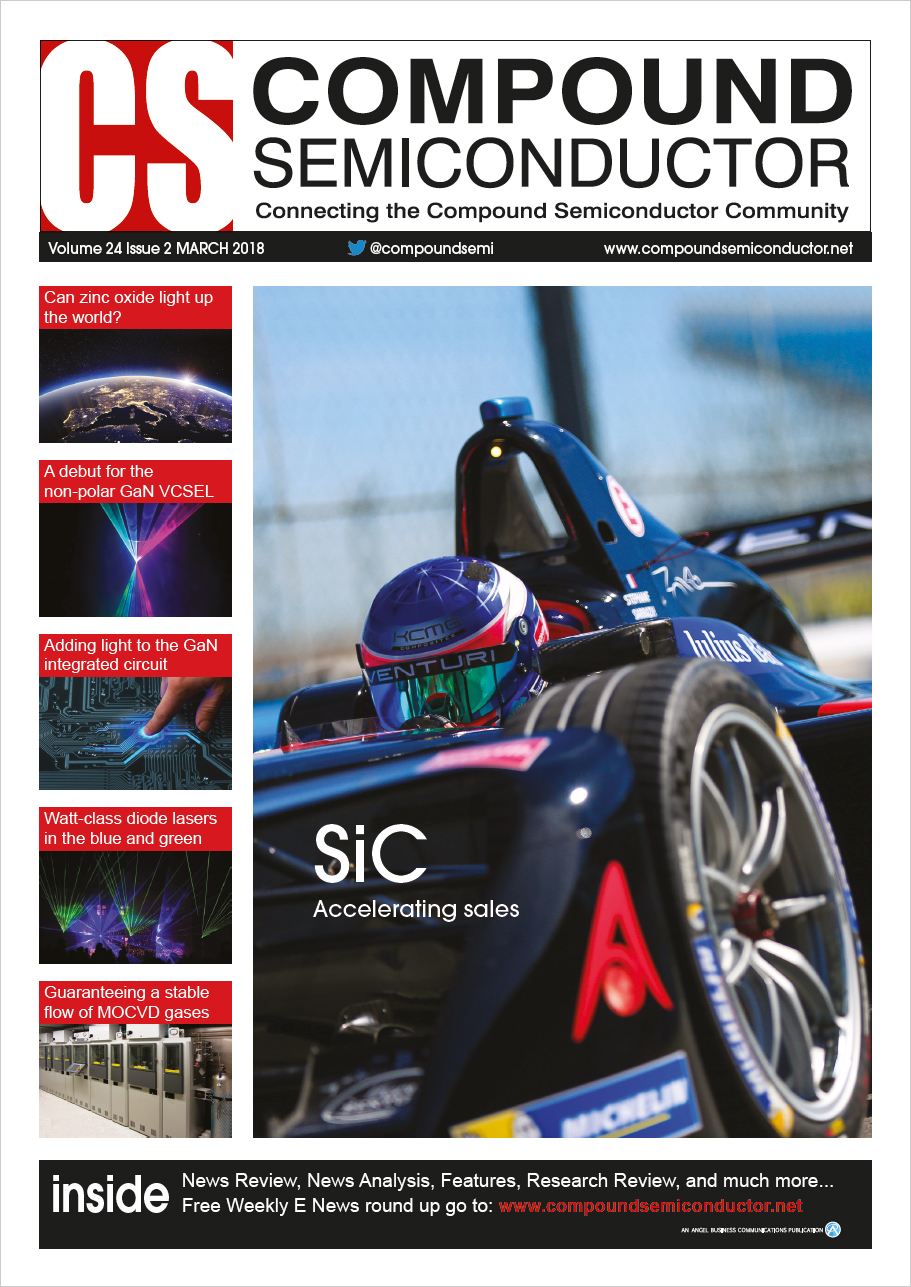
Can ZnO light up the world?
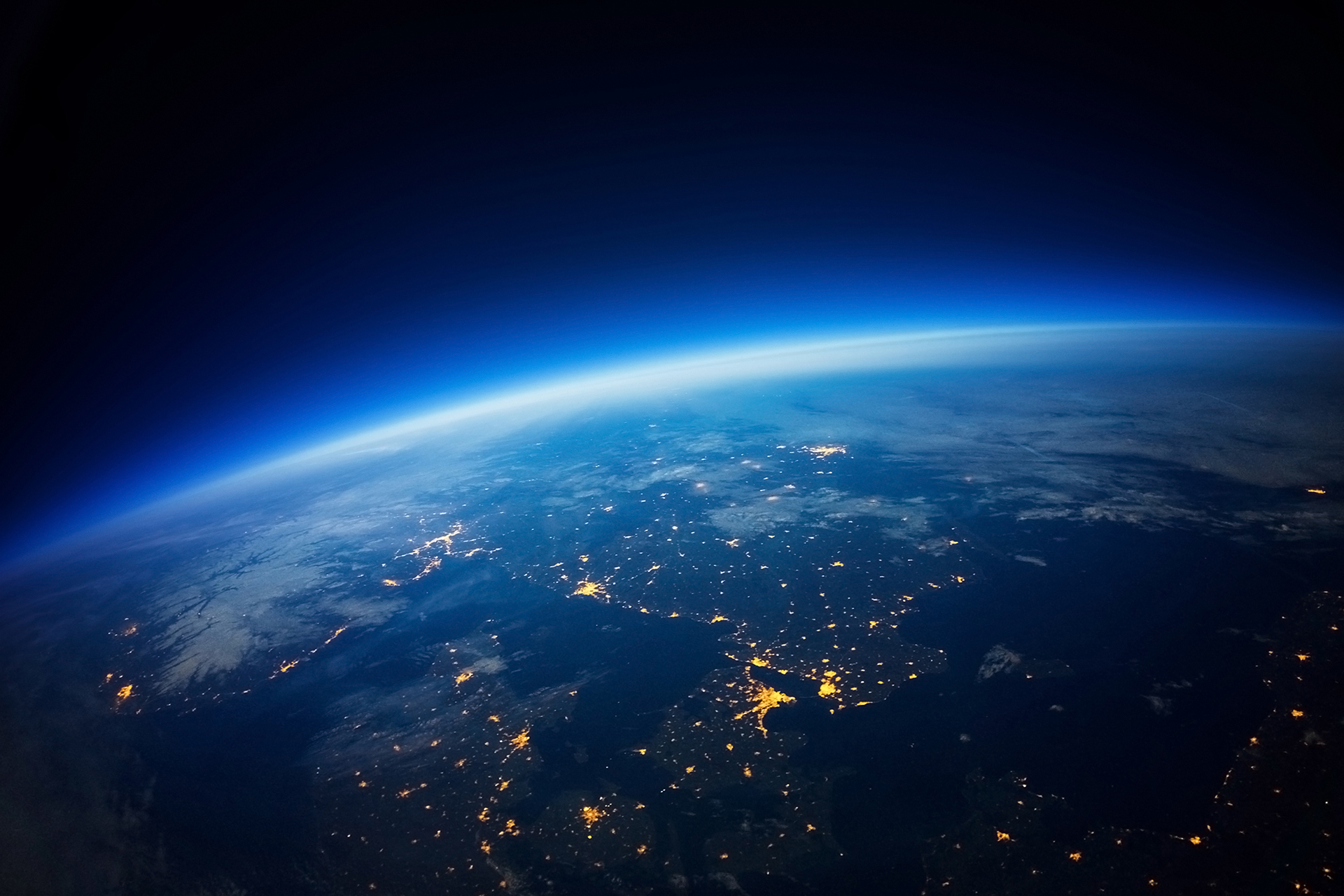
Will it be possible to overcome doping issues in ZnO, and unleash the potential of LEDs and lasers made from this material system by Faiz Rahman from Ohio University
GaN has provided the foundation for the success of blue lasers, blue LEDs and, by extension, white LEDs as well. It is this material system that is underpinning the success of entire solid-state lighting industry of today.
There is little doubt that GaN will retain its role as the de-facto material for generating blue, violet and long-wavelength ultraviolet radiation. Its technology, from both a material and process standpoint, has matured to a point where GaN-based light-emitting devices have become commodity products "“ widely available and very affordable. While transforming the lighting industry, they have undergone two decades of refinement, and now, by widespread consensus, they have reached the pinnacle of perfection. In the coming years, further improvements will be realised in the various metrics that characterise these optoelectronic devices. However, the resulting performance gains will be, at best, very modest. What's needed to take the performance of solid-state devices to an entirely new level is a new material system, possibly accompanied by new device architectures.
That's a route that has often been taken within the semiconductor industry. A study of its history showcases the transformation in performance that can be realised with new materials. Back in the early 1960s, the microelectronics industry made great headway when switching from germanium to silicon. And more recently, further progress has resulted from the emergence of GaAs as a semiconductor of choice for building extremely fast devices.
It is possible that a similar development could take place in optoelectronics, by replacing GaN with a II-oxide compound semiconductor: ZnO (see figure 1). Its potential for making semiconductor lightemitters that outperform those made from GaN has been known for several decades. However, unlocking its promise and realising practical ZnO-based devices has been far from easy. In the remainder of this article, we shall consider why that is the case, review the present status of development of ZnO-based LEDs, and examine the challenges that remain to develop commercially viable devices.
GaN versus ZnO
The best place to start is to compare ZnO and GaN "“ and to focus on the properties that are relevant to making high-performance LEDs. Roughly speaking, ZnO has superior optoelectronic properties to GaN, but it is impaired by its mechanical characteristics (see Table 1). These differences account for the lovehate relationship researchers have had with ZnO for more than two decades.
One key difference between these two wide bandgap materials is that GaN is harder and less susceptible to point defects, while ZnO is soft and prone to defects, such as vacancies and interstitials. This characteristic of ZnO is easy to see when in either powder or crystal form, and heated to temperatures that are high enough to change its colour from white to yellow. This thermochromic change is due to an increase in oxygen vacancies.
ZnO has the upper hand when it comes to exciton binding energies. In this oxide the exciton binding energy is very high, enabling brighter, more efficient light emitters. Another string to its bow is its lower refractive index, leading to a reduction in light entrapment within the device.
Additional, noteworthy advantages of ZnO include the availability of bulk wafers (see figure 2), ease of etching "“ both dry and wet "“ and simple techniques for producing n-type (donor-doped) material. What's more, a ZnO LED can be constructed entirely from this material "“ that is, the substrate, buffer, n- and p-type layers, the active recombination layer and the current spreading layer can all be made of ZnO. This is not the case with GaN LEDs.
Figure 1. Lattice structure of hexagonal (Wurtzite) ZnO, showing Zn2+ ions (red) and O2- ions (blue). This structure can be regarded as composed of Zn-centred and O-centred tetrahedra, as shown.
Taken together, the material advantages of ZnO can simplify device processing, and can contribute to low-cost production of ZnO-based electronics and optoelectronics. LEDs made from ZnO have the potential to be cheaper than their GaN cousins, and outperform them, while ZnO laser diodes promise to exhibit narrower line widths and a lower threshold current. And last but by no means least, ZnO could provide an easier, cheaper route to making mid- and deep-UV light-emitting devices "“ an area where III-nitride devices have not been particularly successful to date.
Doping challenges
The potential performance advantages of ZnO over GaN have spurred a substantial research effort into the development of LEDs made from this oxide. Several aspects of ZnO growth and process technology require further work, but the show-stopper is establishing a method to make stable, hole-rich ZnO. Due to this weakness, most attention has been directed at developing techniques for effective p-type doping of ZnO.
Those working in the area will be aware of two rules of thumb: the wider the bandgap, the harder it is to dope; and p-type doping is more challenging than n-type doping (ZnTe is the notable exception, being easier to dope p-type).
Table 1. Properties of ZnO and GaN
ZnO adheres to both these trends, and it is much easier to dope with donors than acceptors. This limitation hampered the development of GaN devices, until practical techniques for preparing p-type GaN were developed in Japan. History appears to now be repeating itself with ZnO.
For this oxide, p-type doping is not only hampered by a wide band gap. There is also the impediment that when ZnO is undoped, it exhibits n-type conductivity, arising from the presence of naturally-occurring defects. The most notable of these, which are easily formed, are oxygen vacancies "“ that is, oxygen atoms that are not sitting in their usual positions on the crystal lattice. These defects give rise to donor energy levels close to the conduction band edge of ZnO. The intrinsic n-type behaviour that results leads to a substantial electron-mediated conductivity. It is so great that it allows ZnO and lightly aluminiumdoped ZnO to be used as transparent conductors in applications such as transparent touch screens.
If a technique for the p-type doping of ZnO is to be viable, it must counter the material's natural n-type character and flood it with holes. Almost all the conceivable approaches have been tried, including spin-on doping, gaseous diffusion, ion implantation and plasma-phase doping (for a good review that provides a starting point for understanding the contemporary material challenges with the ZnO family of materials, see the paper by Kozuka, Tsukazaki and Kawasaki, given in the Further Reading section).
Obvious starting points for trying to realise p-type ZnO are to substitute group-I elements for zinc sites, or to substitute group-V elements for oxygen sites. However, despite much effort in this direction, it is still very tricky to realise acceptor-doping in this oxide semiconductor. Controlling the doping level is very challenging, because there is the need to compensate for the native n-type character before generating any holes. Complicating matters even further, the hole population of ZnO is drained by the continuous formation of additional electrically-active defects and defect complexes.
Hopes were raised when nitrogen-doped ZnO appeared to be a very promising candidates for creating p-type ZnO epilayers. However, this form of doped ZnO failed to fulfil its early promise. Nitrogendoped material was found to be unstable at higher temperatures, and nitrogen atoms were found to suffer from limited dopant solubility in ZnO. But the good news is that while it is difficult to achieve very high hole concentrations, material with a hole number density of around 1018 cm-3 can be prepared without much difficulty.
A parallel issue is maintaining a stable hole population. Often, the number of holes diminishes over time, due to thermodynamics. Defect complex formation takes place, gradually draining p-doped ZnO of extrinsic charge carriers, and robbing any device of the holes that it needs to deliver its potential operating efficiency.
Finally, it is often a challenge to realise an ohmic metal contact to p-type ZnO. All devices require low specific resistance ohmic contacts, and ZnO throws up its own challenges, which can only be solved through proper optimization of contact metallization.
In short, if ZnO is to work as a feasible material for p-n-junction-based devices, commercial techniques must be developed that can produce a relatively large concentration of holes in ZnO that is stable over time "“ and accessible through practical metallization schemes.
ZnO and GaN?
Efforts at developing ZnO LEDs can be sub-divided into those associated with developing homojunction LEDs, and those pioneering heterojunction variants. Note that the term "˜homojunction' is not applied that strictly within the ZnO comunity: although these LEDs have ZnO-only p-n junctions, they may contain ternary and even quaternary alloys, such as MgZnO and BeMgZnO, to make very high quality charge confinement structures. Meanwhile, heterojunction LEDs are defined as those that pair ZnO with another suitable family of semiconductor materials "“ usually the nitrides, in the form of GaN. One of the merits of combining n-type ZnO with the likes of p-type GaN is that it sidesteps the issue of poor p-type doping in ZnO, to create a promising p-n junction across a dissimilar material boundary.
Over the years, many academic and corporate research labs have demonstrated both homojunction and heterojunction LEDs. They have typical diode current-voltage characteristics, with forward voltage drops of around 4 V. Initially, these LEDs were only capable of a weak output at cryogenic temperatures, but now they can produce good optical output power at room temperature.
Like their GaN cousins, homojunction ZnO LEDs are formed by MOCVD. By incorporating various ZnO-based alloys, emission can be tuned from 350 nm to 420 nm, but output is the most intense at around 360 nm. Note that a weak broad yellow-green luminescence is often seen in these devices, attributed to background defects.
Light emission from an experimental homojunction ZnO LED. The central most luminous region appears white due to overexposure.
Comparatively more effort has been devoted to fabricating heterojunction ZnO LEDs. Pairing ZnO with GaN makes a lot of sense, given that both have hexagonal structures, and their lattice constants differ by only 1.8 percent. These heterojunction devices are actually easier to fabricate, because they can be grown by techniques such as pulsed laser deposition and RF magnetron sputtering.
However, these devices are not fulfilling their promise. Emission often emanates from the non-ZnO component, defeating the purpose of drawing on the superior excitonic properties of ZnO. Nevertheless, these efforts have been useful, because building ZnO LEDs from dissimilar materials has taught the device makers a great deal about carrier confinement and carrier dynamics in ZnO-based devices.
Commercial, hydrothermally-grown, un-doped ZnO wafers.
Another type of ZnO-based LED that has been developed is that based on nanowires. This class of devices promise far better carrier and photon confinement; resulting in more efficient operation. Techniques such as hydrothermal growth and spray pyrolysis have yielded LEDs with an active region for carrier recombination that consists of a dense agglomeration of ZnO nanowires with diameters typically between 100 nm and 200 nm.
Getting the light out
When LEDs are made from wide bandgap materials, such as GaN and ZnO, their high refractive indices lead to the trapping of a substantial proportion of light within the device. To make the LEDs brighter, the confined light must be extracted "“ this is often accomplished by roughening the top surface of the chip.
Figure 2. Typical photonic crystal structure etched on GaN surface. Very similar structures can also be fabricated on ZnO surfaces.
A similar approach, yielding better results, involves etching a regular pattern of shallow holes on the emitting face of an LED. This structure, known as a photonic crystal (see Figure 2), can extract a large proportion of the light that would be total internally reflected within the LED.
For commercial GaN LEDs, the brightness is often boosted by surface roughening and the introduction of photonic crystals. ZnO, if anything, it is even more amenable than GaN to surface structuring for the fabrication of light extracting structures. The ease with which this oxide can be etched makes it very simple to produce rough texturing and photonic crystal structures on ZnO LED surfaces, further enhancing their brightness.
The promise of ZnO as an optoelectronic material is not limited to LEDs, but extends to blue, violet and ultraviolet laser diodes. Making short wavelength semiconductor lasers is not easy, but the higher exciton binding energy found in ZnO can be a major asset. Thanks to this, ZnO semiconductor laser diodes can be brighter and more efficient than their GaN counterparts. Furthermore, they have the potential to be low threshold devices, operating with low power consumption.
Due to more exacting requirements on materials for making diode lasers, ZnO-based lasers trail their LED counterparts. However, once LEDs based on ZnO make their commercial debut, lasers will not be far behind.
So what are the prospects for ZnO LEDs and lasers? While progress has been slow, it cannot be disputed that a great deal of work over the years has led to a much better understanding of doping dynamics in this material. This progress, combined with a favourable commercial outlook, is sustaining the research momentum. Armed with this knowledge, devices could hit the market within a few years, once an industrially-accepted method for p-type doping is in place. Commercialisation is also hampered by longevity issues, which result from poorly understood carrier dynamics in ZnO. Address this with further research, and the dawn of the launch of ZnO bipolar devices will be in sight.