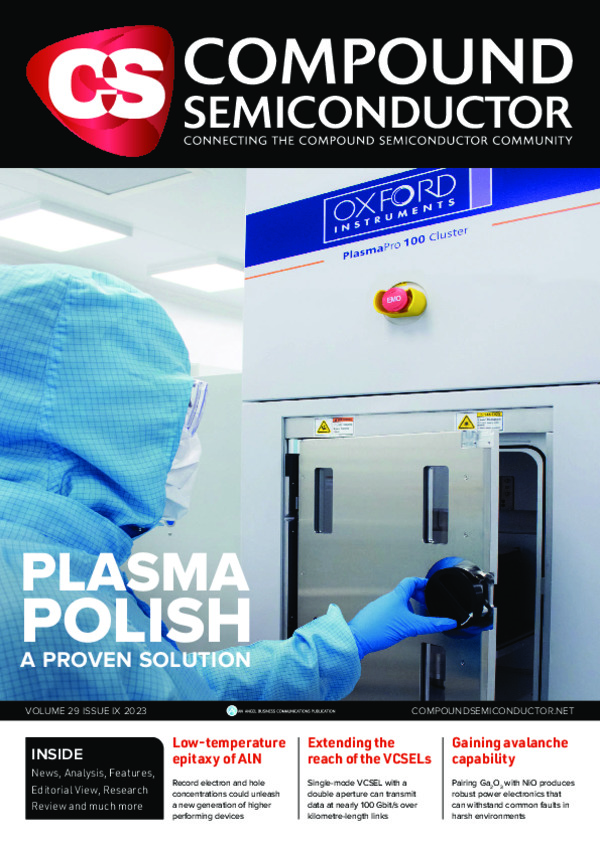
Elevating ethernet networks with distributed-feedback lasers
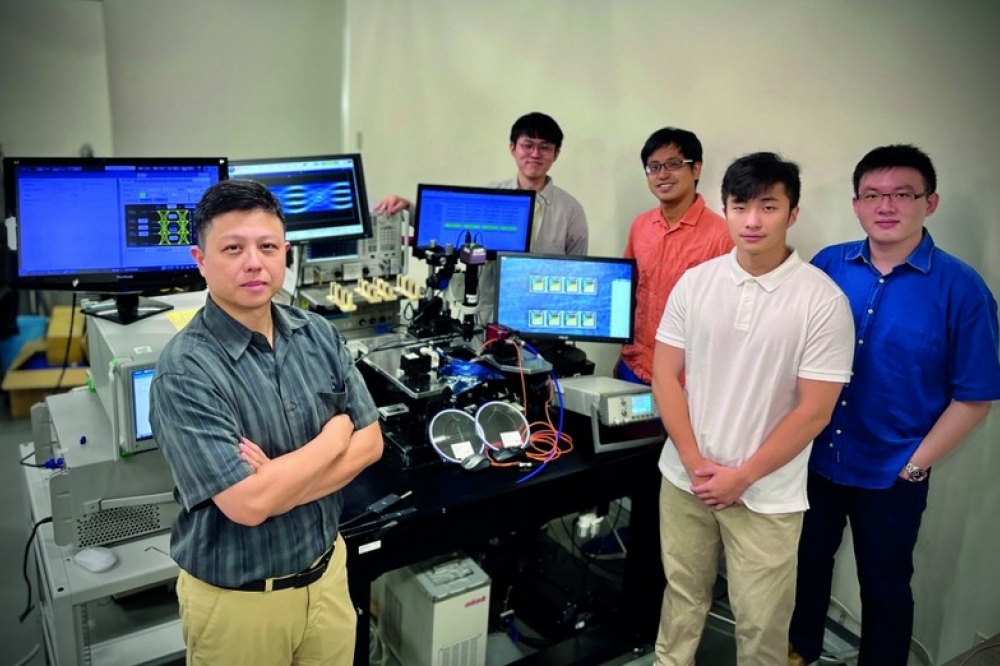
Directly modulate AlInGaAs distributed-feedback lasers provide a low-cost, high-speed source for tomorrow’s Ethernet.
BY TE-HUA LIU, SUNG-PU YANG, HAO-TIEN CHENG, YUN-CHENG YANG AND CHAO-HSIN WU FROM NATIONAL TAIWAN UNIVERSITY
The semiconductor laser has come an awfully long way since its invention in the 1960s by groups at General Electric and IBM. Amongst the many successes of this seminal invention, now a cornerstone of modern technology, is the evolution of today’s optical communication. Serving in diverse domains, from data centres to lidar, this efficient light source is facilitating advances in Ethernet networks, 5G communication, and AI infrastructures. Its users are demanding faster speeds and lower power consumption, driving improvements to its performance.
In the dynamic field of optical communication, manufacturers of lasers are striving to meet future demands. A ubiquitous component in this market is the optical transceiver, key to data transmission and reception. Utilising fibre optic technology, optical transceivers adeptly transform electrical impulses to optical signals and vice versa. In the 5G era, their role is indispensable, especially in front-haul and back-haul connections. Data from 2022 reveals the 25 Gbit/s transceiver’s dominance, accounting for over 30 percent of the optical communication market’s revenue. With the evolution of Ethernet, suppliers are pressed to address growing data traffic, prompting innovations in transmission speeds.
Figure 1. An illustration of the 1.31 µm directly modulated AlGaInAs-based ridge-waveguide distributed-feedback laser.
To support an increase in data traffic, driven by the increasing use of smartphones and cloud computing, there has been an introduction of 100 Gigabit Ethernet (100 GbE). Here, the emphasis is on transceivers that transmit data over long distances, maintaining network stability. However, increasing the transmission distance is far from trivial, due to chromatic dispersion within the optical fibre.
One solution is to use lasers that emit in a spectral window centred around 1.31 µm, a domain that ensures low chromatic dispersion. To produce a narrow emission profile, such lasers incorporate an intra-cavity grating structure. Operating at 25 Gbit/s and using four wavelength channels, these sources are apt for mid-to-long-range communication.
The ever increasing demand for data is driving advances in transceiver capability, with industry migrating towards 100 GbE and 400 GbE systems, succeeding the current 10 GbE and 25 GbE models. Discussions surrounding standardised transmission rates continue, and potential 400 GbE configurations include 16 x 25 Gbit/s, 8 x 50 Gbit/s, and 4 x 100 Gbit/s.
What’s clear is that network operators can either increase their capacity or reduce their channel count by turning to transceivers that operate at faster data rates. The two standout laser designs that could be deployed in these transceivers are external-modulation lasers and direct-modulation lasers. There is a transmission prowess that comes from external modulation, associated with data transmission over long distances, but this comes with a higher purchase price. As well as lower cost, the direct modulation laser is compact and efficient, making it the top choice for multi-channel integration.
Figure 2(a). Light-current-voltage (L-I-V) characteristics of a 1.31 mm
directly modulated AlGaInAs-based ridge-waveguide distributed-feedback
laser across a wide temperature range of 25-85 °C (b). Characteristics
of optical spectra at different temperatures for laser operation at 50
mA (d). Electro/optical (E/O) response of the laser operated at 60 mA
across 25–85 °C.
Directly modulated lasers
The merits of the directly modulated distributed feedback laser have motivated our team at National Taiwan University to improve this device while drawing on our robust domestic supply chain. We have made a strategic choice to employ AlGaInAs as the foundational material, due to its pronounced conduction band offset. It’s a selection that ensures that the carriers remain within the laser diode’s active region, preserving optoelectronic integrity, even under strenuous conditions such as elevated temperatures or currents.
Our architecture incorporates a ridge waveguide structure. Using this design, we sidestep additional regrowth procedures during manufacturing, thereby gaining on two fronts: we bring down production costs, and we improve device reliability.
Crafted on an n-type doped InP substrate with the assistance of MOCVD technology, our laser has an AlGaInAs active zone surrounded by a separate confinement heterostructure. This technology features a meticulously designed grating, formed from alternating layers of InGaAsP and InP, and a cavity precisely engineered at 150 µm (see Figure 1). To optimise resonance, we apply anti-reflective and high-reflectivity thin films to the front and rear facets, respectively.
During the transceiver’s operation, its internal module temperature escalates. It’s important that when this happens, the laser upholds its peak optical power and maintains its wavelength uniformity and adequate modulation frequency. All these factors are instrumental in determining the transceiver’s efficacy.
To thoroughly gauge our laser’s attributes, we have carried out DC and microwave evaluations. These investigations reveal that our AlGaInAs-based ridge-waveguide laser consistently performs across an extensive temperature spectrum (see Figure 2, which shows temperature-variant tests that evaluate optical power, spectral attributes and electro-optical responses). What stands out is the spectrum’s minimal drift – there’s just a 4.5 nm wavelength deviation between ambient temperature and 85 °C.
We are particularly encouraged that even within this temperature bracket, the disparity in intensity between the primary mode and its adjacent side mode is always greater than 50 dB. This asset highlights our laser’s superior single-mode characteristics. For long-haul transceivers covering expanses of several kilometres, this attribute is incredibly valuable, as any deviation towards multi-mode behaviour threatens to heighten transmission discrepancies. The steadiness of the optical mode and its wavelength are paramount for optimal functionality of laser diodes within optical transceivers.
Electro-optical microwave tests determine a 3 dB frequency around 21 GHz at 25 °C for our laser. This device retains a frequency above 15 GHz, even when the temperature reaches 85 °C. Delving into the electro-optical response metrics reveals that our laser exhibits performance capabilities beyond 30 Gbit/s at 25 °C and remains robust, delivering above 20 Gbit/s at 85 °C.
Figure 3. Eye diagrams of a 1.31 µm directly modulated AlGaInAs-based
ridge-waveguide distributed-feedback laser at room temperature,
showcasing 35 Gbit/s and 30 Gbit/s data rates under a modulating signal
of 215-1 NRZ pattern.
Our assessments, visually represented with eye diagrams (see Figure 3), indicate that our distributed feedback laser is adept at realising data transmission speeds surpassing 35 Gbit/s while operating in a Non-Return-to-Zero (NRZ) mode with a signal sequence of 215-1. Notably, this transmission efficacy is attained without the need for specialised techniques, such as pre-emphasis or equalisation. Our illustrative eye diagrams underscore our laser’s promising potential, hinting at its capability to facilitate 100 GbE and 400 GbE transceivers when integrated across multiple channels.
By capitalising on the outstanding DC and high-frequency transmission capabilities of our laser, even at increased temperatures, there is the promise that we might be able to produce ridge-waveguide distributed-feedback lasers that are free from active cooling. Success in this regard would pave the way for a reduction in power consumption and curtail costs associated with thermoelectric cooling components inside transceivers.
Driving commercialisation
Our successful development of AlGaInAs-based ridge-waveguide distributed-feedback lasers offers another route to advancing data transmission, and making global information networks faster and more reliable. Since the inception of semiconductor lasers, every generation of scientists and engineers has contributed to the evolution of this technology. The emergence of our AlGaInAs-based laser brings new prospects for high-speed optical communication.
Superior characteristics – including stable light output under varying temperature conditions, a single-mode spectrum, high-frequency capability, and flexibility for multi-channel integration – position this device as an exceptional candidate for high-capacity Ethernet networks.
Now more than 60 years since their invention, semiconductor lasers have permeated various corners of modern life. Whether in sensing or communication applications, their significance is unquestionable. Given the diverse array of applications, system providers expect prompt responses from laser suppliers. So, to address this urgent need, our team has established a technology start-up, LiVe Optronics Co., Ltd. We anticipate that through this venture we will translate years of research experience into tangible products, rapidly designing semiconductor lasers for customer systems and producing devices through international collaboration. Through LiVe Optronics, we also expect to foster more collaborative relationships and accelerate the development of semiconductor lasers.
Main image: The team from National Taiwan University, alongside their designed semiconductor laser devices. From left to right: Chao-Hsin Wu (the PI), Te-Hua Liu, Hao-Tien Cheng, Yun-Cheng Yang, and Sung-Pu Yang