Boosting Brightness With V-Shaped Pits
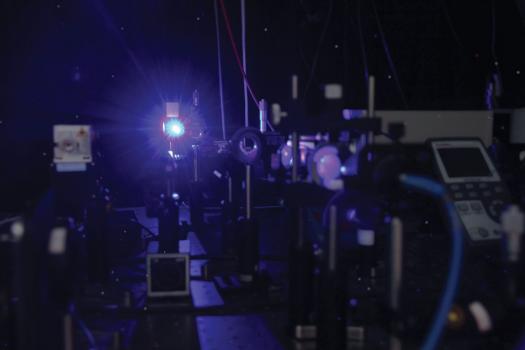
Optimising V-shaped pits combats droop by preventing carriers from disappearing into non-radiative threading dislocations
BY CHIAO-YUN CHANG, HENG LI AND TIEN-CHANG LU FROM THE NATIONAL CHIAO TUNG UNIVERSITY
Talk of a looming energy crisis is fuelling efforts throughout the world to trim carbon dioxide emissions and energy consumption.
There are many opportunities that are available for succeeding on both these fronts, including the uptake of efficient lighting. This could make a big difference: According to the US International Energy Agency, in 2007 illumination accounted for 20 percent of global electricity consumption, and while this figure may have fallen since then, it will still be significant.
Recently, lighting has undoubtedly become more efficient, thanks in part to the banning of the sale of the incandescent bulb in many countries. Fluorescents are now the biggest seller, but sales of solid-state sources are rising fast, spurred by the great attributes of the LED: It is free from mercury; it has a small foot print; its electro-optical conversion efficiency is very high; and it lasts for many years.
Since the first GaN-based LED emerged out of the labs in the 1990s, the efficiency of this source has rocketed. By 2010, the GaN-based white LED, which is formed by combining a blue LED with fluorescent powders, could produce more than 150 lm/W and operate with an electro-optical conversion efficiency of 60 percent. And since then, even higher figures have been reported.
However, there is still work to do. If higher efficiencies can be reached, this will reduce energy consumption. And if the LED can deliver this efficiency at a far higher current density, then fewer chips will be needed to build the light source, making it a cheaper and more attractive purchase.
Delivering high efficiencies at high current densities is very tough, however. That's because a mysterious malady known as droop is wreaking havoc with LEDs, causing emission efficiency to plummet as the current through the chip is cranked up. A detailed understanding of its origin would help to address this, as it could aid the design of droop-busting LED architectures.
Given the importance of droop, it is of no surprise that its cause is hotly debated, with the research community offering a wide range of possible theories. Explanations that have been proposed include those involving: the polarization field that results from the quantum confined Stark effect (QCSE); carrier overflow; non-uniform carrier injection and distribution within the active region; and Auger recombination.
One topic that has not received that much attention, however, is the role that defects play in droop. Growing the epitaxial layers on a native substrate minimizes these imperfections, while diminishing droop at very high current densities. However, GaN substrates are very expensive. Consequently, the vast majority of today's LEDs are formed on a foreign substrate. Sapphire is widely used, despite its significant lattice and thermal-expansion mismatches with GaN that give rise to a high density of threading dislocations, typically ranging from 108 "“ 1010 cm-2. Reductions in the defect density are possible by turning to growth on GaN templates formed by epitaxially lateral overgrowth or HVPE, but the penalty to pay for both these approaches is a relatively high fabrication cost.
Figure 1. Top-view, scanning electron microscope images of InGaN/GaN multi-quantum wells that reveal the formation of V-pits on the templates of (a) 10 pairs (b) 15 pairs (c) 30 pairs and (d) 60 pairs of an InGaN superlattice. These images, taken at a magnification of 3000, reveal that the average diameters of V-pit sizes in the InGaN multiple quantum wells with 10 to 60 pairs in the superlattice are 170 nm , 220 nm , 280 nm and 370 nm, respectively.
To reduce threading dislocations and enhance photon extraction efficiency, today's GaN LEDs are manufactured on patterned sapphire. As a result, epitaxial layers are better, but they are still riddled with defects: Threading dislocation densities are typically in the range
107 "“ 108 cm-2, which is equivalent to having one of these dislocations in every micron square.
Since the diffusion length of carriers is on the order of a micrometre, it would be a gross oversight to neglect the influence of threading dislocations on the luminescence efficiency. It is well known that these dislocations have a strong influence on carrier leakage paths and trap centres. They are also responsible for the dark areas "“ that is, areas with relatively low emission efficiency "“ that have a radius comparable to the carrier diffusion length. These regions result from carriers diffusing into non-radiative, deep-level states associated with threading dislocations.
V-shaped pitsOne of the consequences of threading dislocations is that they lead to the formation of V-shaped pits within the GaN-based LED. These imperfections, known simply as V-pits, are hexagonal, and they form at an open core at the apex of threading dislocations, with facets inclining to six sidewalls on (1011) planes at an oblique angle of approximately 60Ëš.
An awareness of these V-pits is not new "“ as far back as 1997, Andreas Hangleiter's group at the University of Stuttgart found that threading dislocations could easily induce the formation of V-pits during the growth of InGaN multiple quantum wells for the active layer of LEDs. These V-pits would form more easily when reducing the temperature for the growth of the active region, or when increasing the indium content in the quantum well. It has been suggested that the formation of these pits is related to indium segregation on the (1011) surfaces around the dislocation core.
A key characteristic of the V-pit is that its growth rate on the sidewall of the quantum wells along the (1011) plane tends to be slower than that on the c-plane, which is the plane of the epitaxial film. The slower growth rate results in a reduction in the thickness of the wells on the inclined V-pit semi-polar planes, and an emission of photons with higher energies. The latter phenomenon has been seen in the emission spectra of LEDs that were scrutinised by Andreas Hangleiter's group from the Technical University of Braunschweig (results were reported in 2005).
Narrowing the quantum well thickness is good news, because it creates an energy barrier that can prevent carriers from entering the luminescence-quenching threading dislocations. This offers an explanation for the relatively high emission efficiency of InGaN LEDs with such a high defect density.
In 2014, a group from South Korea, led by researchers at Samsung Advanced Institute of Technology, observed this carrier-blocking capability of V-pits with different barrier heights. The team employed a one-dimensional model, using a k.p ap proximation to determine how droop is influenced by the height of the energy barrier created by V-pits. Calculations revealed that higher-energy barriers can combat droop by decreasing the likelihood of tunnelling at high-injection currents. What's more, the presence of inverted hexagonal pyramids could help light scattering; and it could aid the electrical characteristics of InGaN LEDs, by hindering magnesium incorporation of p-type GaN in the capping on top of V-pits. This reduced magnesium incorporation would increase local resistance, and thus resist carrier transportation to the threading dislocations.

Figure 2. Cross-sectional transmission electron microscopy images of V-pits for InGaN multi-quantum wells with (a) 10 pairs (b) 15 pairs (c) 30 pairs and (d) 60 pairs of InGaN superlattice layers. Increasing with the V-pit size is the thickness of sidewall multi-quantum wells, which are estimated to be 1.14 nm, 1.16 nm, 1.23 nm and 1.35 nm on semi-polar planes for 10, 15, 30 and 60 pairs of InGaN superlattice layers, respectively.
While other groups have shown that the formation of V-pits can aid LED performance by undermining the impact of threading dislocations, the quantitative impact of the V-pit structures is still unclear.
To address this shortcoming, our group at National Chiao Tung University in Taiwan has undertaken a systematic investigation of the relationship between the emission efficiency of InGaN/GaN quantum wells and the nanoscale structure of V-pits along threading dislocations.
An important aspect of this work has been the use of embedded InGaN/GaN superlattices with low indium composition under the active region. Inserting these superlattices under quantum wells assists the formation of V-pits along the threading dislocations. Their size can be adjusted by varying the number of superlattice pairs.
We have studied V-pits by examining our samples with electron microscopes and measuring the photoluminescence they produce. A quick glance at a top-view SEM image of the V-pits on our quantum wells suggests that they are distributed randomly (see Figure 1). However, that is not the case, with spatial frequency mapping after Fourier transformation revealing hexagonal lattices related to the underlying patterned sapphire. In other words, the V-pits are highly correlated to the substrate. Their density is similar in all our samples, and is typically 1.7 x 108 cm-2.
Our TEM offers another insight into the defects in the samples (see Figure 2). Images reveal that threading dislocations extend along the c-direction, originating from the flat c-plane region of patterned sapphire; and that the formation of V-pits is triggered by the termination of threading edge dislocations. The V-pits start to appear on the surface of the superlattice, and their size increases with the number of pairs in this heterostructure. And as the size of the V-pit increases, there is a gradual increase in the growth rate of the sidewall of the quantum-well region.
Our samples, which have superlattices ranging from 10 pairs to 60 pairs, have been subjected to power-dependent photoluminescence measurements at 10K. At lower excitation powers, light emission came predominantly from planar quantum wells, but at higher powers an extra shoulder peak appears, due to emission from the narrow sidewall wells of V-pits.
As the V-pits get bigger, they provide a smaller barrier to carriers entering the non-radiative recombination centres of the threading dislocations. Consequently, in general the samples with the bigger V-pits have a lower internal quantum efficiency (see Figure 3(c) and (d)).

An insight into droop is provided by the normalized photoluminescence emission efficiency from our range of InGaN quantum wells (see Figure 3(b)). The efficiency droop kicks in at the same time as the onset of the emission from the sidewall quantum wells on V-pits. At high excitation powers, these hot, high-energy carriers are more likely to jump over the energy barriers around the V-pits, leading to more pronounced droop of the photoluminescence. One way to reduce droop, therefore, is to introduce a superior V-pit structure.
One peculiarity associated with our results is that it is the InGaN quantum wells above the 10-pair superlattice that have the lowest value for the internal quantum efficiency, despite having the smallest pit size and the largest energy barrier. This abnormality shows that the barrier energy of the V-pit cannot, on its own, account for the relationship between quantum efficiency and V-pit size.
To develop a better understanding of the influence of threading dislocations and V-pits on the photoluminescence spectra of our range of samples, we constructed a two-dimensional model for the spatial carrier distribution. This model drew on the carrier rate equation and the diffusion effect, by carefully considering the diffusion length, carrier generation rate and carrier life time.
According to this model, the emission intensity gradually decreases near the non-radiative recombination centres of threading dislocation (see Figure 4).
Figure 3. (a) Photoluminescence emission spectra of InGaN multi-quantum wells with 370 nm V-pits at excitation powers of 5 mW and 20 mW at 10K. (b) The normalized efficiency of the photoluminescence emission for InGaN multi-quantum wells with different V-pit sizes as a function of excitation power. (c) The emission peak energy of sidewall InGaN multi-quantum wells as a function of V-pit size at the temperature of 10K and the high excitation power. (d) The internal quantum efficiency of InGaN/GaN multi-quantum wells with varying V-pit size.
Our calculations also show that the benefit of a higher barrier results from smaller V-pits can be overshadowed by the shorter diffusion distance between the centre of the threading dislocation and the V-pit boundary. This explains why the active region above the 10 period superlattice did not produce the brightest emission.

Figure 4. The two-dimensional distribution of the emission intensity for InGaN multi-quantum wells with the presence of V-pits and threading dislocations.
Our work offers another lever for manufacturers of LEDs that are looking to minimise droop: Control the formation of V-pit structures, which can provide a barrier to carriers entering non-radiative threading dislocations. This approach is hopefully a stopgap, however, that will be superseded by the growth of low-defect density materials. Whether this is practical will depend on cost, and it may be that the control of V-shaped defects is not just used for blue LEDs, but their green and ultraviolet cousins too.