Optimising LEDs for wireless communication
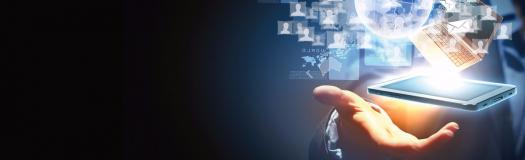
BY JONATHAN MCKENDRY FROM THE UNIVERSITY OF STRATHCLYDE
WE ARE CONTINUING to refine the way we use light to transmit information. Back in the ninth century, during the Arab-Byzantine wars, communities would relay very simple messages by the lighting of bonfires on a particular hour of the day. Fast-forward to the 1860s, and communication at a rate of several words per minute could be transferred between sailors on-board ships of the British Royal Navy using a form of Morse code. And a decade or so after that, the well-known scientist, engineer and inventor Alexander Graham Bell demonstrated a "˜photophone', a device that used an intensity-modulated beam of sunlight to transmit speech from one location to another.
Today, there is interest in transmitting data at far higher rates, using light bulbs as the source. If these bulbs are incandescents or fluorescents, high data rates are out of reach. But with the introduction of LED lighting, transmission at hundreds of Mb/s, or even several Gb/s, is now feasible. As the uptake of solid-state lighting is booming, there is good reason to believe that an era of using white LED sources for simultaneous illumination and wireless communication, often dubbed "˜Li-Fi', is on the horizon.
Various patterns displayed on a micro-LED array. Each pixel is 100 µm by 100 µm and is individually addressable. The red scale bar is 300 µm.
Li-Fi, which is a form of visible-light communication (VLC), involves using LEDs to simultaneously illuminate an environment and transmit data via modulating the intensity of the source. Modulation is performed in a manner than is imperceptible to the human eye and compatible with dimming schemes. One of the great strengths of Li-Fi is that it makes use of a wide, licence-free visible spectrum. It could help to maintain the rapid growth in wireless data traffic, which rocketed from 2.5 Exabytes (Eb) in 2014 to 4.2 Eb in 2015 and is projected to outstrip improvements in existing radio-frequency wireless technology (see Figure 1).
Taking standard "˜off-the-shelf' white-emitting GaN LEDs, a team from the TeCIP Institute in Italy has already demonstrated data transmission rates in free space in excess of 1 Gb/s, and realised 3.4 Gb/s through the simultaneous use of red, green and blue LEDs. However, while the LEDs used for these studies offer high modulation bandwidths in comparison to incandescent and fluorescent sources, they are still relatively modest, typically 10-20 MHz. These low modulation bandwidths limit the maximum data rates, although it is possible to increase this with more complex modulation schemes.
A narrow bandwidth is not, in general, a concern for the designers of LEDs for lighting, as their focus is optimising characteristics such as the luminous flux and wall-plug efficiency. But it does matter to our team from the Institute of Photonics at the University of Strathclyde, which is participating in a four-year project, entitled Ultra-parallel visible-light communications, that kicked off in October 2012. Backed by £4.6 million of funding from the Engineering and physical Sciences Research Council, we are working with colleagues from the Universities of Cambridge, Edinburgh, St. Andrews and Oxford towards the goal of determining how data rates are influenced by novel modulation schemes, receivers, colour-converting materials and LED-based transmitters.
Our particular focus lies on the transmitter side. We are able to leverage our expertise in micro-LED arrays, which contain many individually-addressable LEDs with typical dimensions of less than 100 µm. Thanks to their small size, hundreds of these devices − which can be produced by conventional processing of commercially-available GaN LED wafer material "“ can fill an area that would usually be occupied by a single LED.
Shrinking device dimensions is highly beneficial for data transmission, because the modulation bandwidth is no longer limited by the device's resistance and capacitance "“ instead, it is governed by carrier lifetime. This depends on the carrier density, which can much higher in our LEDs. They operate at typically 15,000 A cm-2, compared to just 10-100 A cm-2 for most standard LEDs. Due to this ability to handle higher current densities, modulation bandwidths are an order of magnitude higher than those for conventional LEDs (see Figure 2)
Figure 2. The modulation bandwidth of three micro-LEDs, each with a peak emission wavelength of 450 nm, at differing operating current densities. Each micro-LED has a different diameter, but are from the same array. This plot highlights that: micro-LED bandwidth is dependent on current density (with the different-sized micro-LEDs showing the same bandwidth when operated at the same current density); and smaller micro-LEDs are able to be operated at higher current densities than their larger counterparts, because they are able to reach higher modulation bandwidths.
We have used our devices to break the record for the highest reported data rate from a single GaN LED. Using a single micro-LED pixel with a peak wavelength of 450 nm and a pixel diameter of 50 µm, we realised a data rate of 3 Gb/s using orthogonal frequency-division multiplexing (see Figure 3).
The signal-to-noise ratio available at the receiver limited the separation of the transmitter and receiver to 5 cm. However, by adding transmitter and receiver optics, this data link can be extended to 10 m. This result highlights the capability of micro-LED optical transmitters for multi-Gb/s data transmission rates over useful link lengths.
Figure 3. The measured bit-error-ratio (BER) "“ that is, the ratio of the total transmitted bits to the total incorrectly-transmitted bits − as a function of data rate for single micro-LED orthogonal frequency-division multiplexing. The red line indicates a BER of 2à—10-3, below which a technique called "˜Forward error-correction' can be used to correct any errors in the bit stream. This comes with a 7 percent overhead penalty, so the ≈3.2 Gb/s point on the figure corresponds to the error-free 3 Gb/s.
Following our demonstration of the potential of individual micro-LED emitters, our focus shifted to exploring the possibilities of utilising multiple pixels within an array. With micro-LED sources, their multi-emitter, pattern-programmable format has the potential for individual addressing of hundreds of emitters. By turning from a single emitter to a multiple variant, data communication can be enhanced in different ways. For example, multiple data streams can be transmitted in parallel to increase the overall data rate "“ a technique known as multiple-input, multiple-output, or MIMO. Alternatively, data can be encoded into the spatial position of the pixels. With this approach, which is a form of spatial modulation, transmission of data is via the switching on of the appropriate LED(s) during designated times.
To investigate increases in data rates via the use of several pixels in an array, we built a scalable, integrated VLC system consisting of a: micro-LED array; CMOS LED drivers; and a 3 by 3 avalanche photodiode array, fabricated with a 0.18 µm CMOS process. With this approach, every micro-LED can transmit identical data (a so-called "˜ganging' mode), in order to increase the power of the transmitted signal. Alternatively, the system can operate in MIMO mode, with each pixel transmitting a separate data stream. Another degree of flexibility is associated with the handling of the output of the detectors in the receiver array, which can be summed together or read out separately. When operating transmitters and receivers in the MIMO mode, we have realised a data rate of 1 Gb/s over a distance of 1 m (see Figure 4)
Another area of our research concerns "˜colour-converters'. These are materials that can be used in combination with LEDs to generate white light − and can also enable devices to generate signals at multiple wavelengths, as this provides an opportunity for wavelength-division multiplexing. The vast majority of commercial white-emitting LEDs generate their broad emission by using a blue LED to pump yellow-emitting cerium-doped YAG phosphor. White light results from the colour mixing of blue and yellow. This approach is an efficient way to generate white light, but the device that results is far from ideal for communication. That's because the phosphor has a very long excited state lifetime "“ it is of the order of microseconds − so it is slow to respond to modulation signals.
Due to this issue, the majority of researchers that are developing VLC systems completely filter out the phosphor emission at the receiver, to leave just the comparatively fast signal from the blue-emitting LED die. Two major downsides result from this approach: A substantial proportion of the optical output of the LED is not used to transmit data, and the yellow component of the emission is not used to realise parallel data transmission at multiple wavelengths.
To address these weaknesses, we are investigating alternative colour-converters. Like conventional phosphors, they can be paired with an LED to create white light, but the critical difference is that they have a much faster response "“ typically, it is in the regime of nanoseconds to tens of nanoseconds. With this approach, the colour-converted emission can be used as a high-speed optical data channel, rather than being discarded, which is the case for LEDs incorporating yellow YAG-based phosphors. Our trials with a blue micro-LED and a yellow-emitting polymer colour-converter have enabled data transfer at 1.68 Gb/s, which is the highest value for VLC for a single white-emitting source.
Figure 4. A custom scalable, integrated VLC system can be constructed from a micro-LED array and an avalanche photodiode array are also shown.
A second option that we are exploring is to unite a GaN device with a membrane of II-VI material with a very fast response time. By capillary-bonding a green-emitting II-VI platelet to an LED, we have created a source with a modulation bandwidth of 145 MHz (see Figure 5). As well as generating white light, these materials could potentially be patterned, enabling the construction of multi-colour, micro-LED arrays. These multi-colour sources could transmit multiple data streams simultaneously over free-space or routed through a waveguide.
While the development of LED lighting will continue to be driven by demand for high-quality, highly-efficient solid-state lighting, the results emerging from our UPVLC project highlight the capability of these devices to deliver very high data rates, once they have been adapted and optimised for communication.

