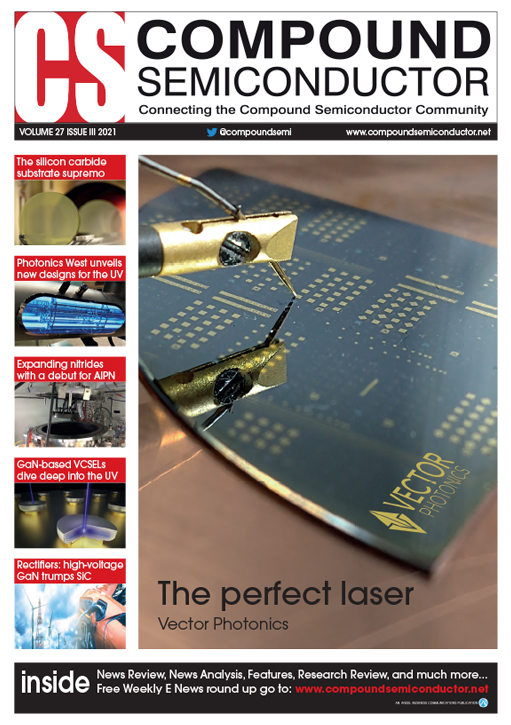
Photonics West: Deep-UV designs
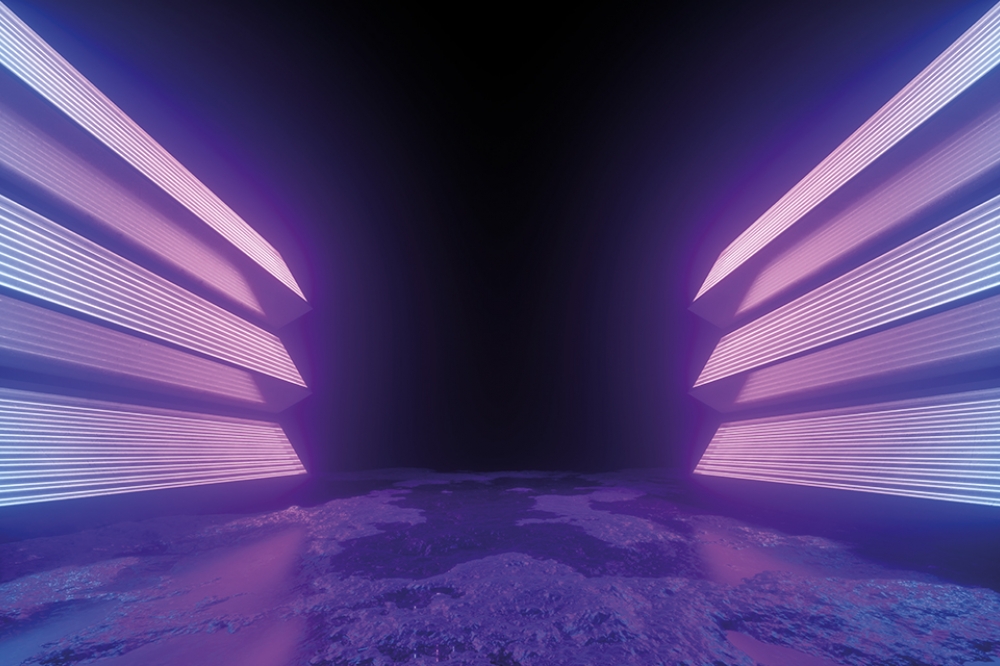
Speakers at Photonics West outlined a variety of options for improving the performance of the deep-UV LED
BY RICHARD STEVENSON
HOW DO YOU VIEW the deep-UV LED? It’s easy to see this source as only a killer of viruses, and in particular Covid-19, now that the pandemic has been dominating our thoughts for more than a year. But the opportunities for this class of LED extend well beyond the purification of air, water and surfaces. This chip can also lie at the heart of systems sensing a range of gases, and provide the light source for non-line-of-sight communication.
For all these applications, the deep-UV LED has its pros and cons. Unlike the more traditional sources of emission in this spectral domain – the excimer lamps, and those based on the likes of mercury, deuterium and xenon – it is not fragile, bulky, or suffering for a short lifetime. But it’s wall-plug efficiency is pitiful, trailing that of its blue cousin by a substantial margin, particularly when emission ducks below 250 nm.
With the deep-UV LED, there are so many barriers to a better performance. However, look at this situation in a more positive light, and you’ll see that there are also so many opportunities to propel the performance to a new level. These options, discussed in detail at this year’s SPIE Photonics West, include: reducing the defect density in the foundation, to prevent the active region from being riddled with a high degree of imperfections; increasing the internal quantum efficiency by improving the architecture of the light-emitting region; and delivering a hike in the proportion of light that exits the chip.
Which foundation?
A compromise is inevitable when selecting the foundation for the deep-UV LED. Bulk AlN minimises the defect density in the heterostructure, but it is pricey and availability limited. Consequently, developers and manufacturers of deep-UV LEDs tend to use a sapphire substrate, and employ growth technologies to drive down the defect densities in the epistructure. Quash defect densities as low as 108 cm-2 and internal quantum efficiency as high as 80-90 percent should be possible, assuming the absence of non-radiative defects.
Over the last few years Michael Kneissl’s group from TU Berlin, Germany, have been working in collaboration within researchers at FBH to increase the material quality in AlN films grown on sapphire. Kneissl, who gave an invited talk at this year’s Photonics West, explained that his group initially focused on growing a thin AlN film on sapphire, etching columns through the nitride and into the substrate, and applying overgrowth that caused AlN to coalesce. However, while this approach formed good films with a thickness of 5.5 μm, it was not ideal. “Patterning is time consuming and costly,” admitted Kneissl.
More recently, his team has turned to a low-cost approach pioneered by Hideto Miyake’s group at Mie University, Japan. This method involves sputtering AlN on sapphire, before annealing the resulting template under nitrogen gas at 1700 °C. “After this three-hour anneal we observe a very significant reduction in threading dislocation density in the AlN layer,” explained Kneissl. These templates have a good morphology, with a threading dislocation density of 7.2 X 108 cm-2 realised in a 350 nm-thick film.
The latest approach by Kneissl and co-workers is to combine both techniques, forming an AlN-on-sapphire foundation by sputtering, annealing and overgrowth. This promises to lead to even lower dislocation densities. Results are encouraging, with samples produced by high-temperature annealing and epitaxial overgrowth providing the highest values for the internal quantum efficiency. Measurements suggest a value of almost 30 percent.
Kneissl said that encapsulated LEDs produced on this template emit at 265 nm, are capable of producing 54 mW at 350 mA, and have a peak external quantum efficiency of 3.7 percent. He believes that far higher values could be realised through the introduction of reflective contacts and a transparent p-side.
Facing together
The trailblazer of the sputtering and annealing approach, Miyake, also spoke at this year’s Photonics West. His team form high-quality templates by sputtering AlN on sapphire and then taking pairs of AlN-on-sapphire epiwafers, placing their growth faces together, and annealing them under nitrogen at 1 atmosphere for between 10 minutes and 10 hours. “Typically we use 3 hours,” added Miyake.
To illustrate the dramatic improvement in crystalline quality wrought by annealing, Miyake presented X-ray diffraction data showing that the elevated temperature delivered a dramatic reduction in the diffraction peak width from 532 arcsec to 49 arcsec.
Efforts to optimise the sputtering conditions have included evaluation of the impact of sputtering pressure, layer thickness and annealing temperature. These experiments showed that when annealing films at 1700 °C for 3 hours, a lower sputtering pressure reduces the chances that cracks form. Reduce the sputtering pressure to just 0.03 Pa and it is possible to produce crack-free films more than 800 nm-thick. When it comes to optimising crystalline quality, the sputtering pressure influences the ideal thickness. For example, when growing at a sputtering pressure of 0.03 Pa, measurements of X-ray diffraction indicate that the ideal AlN thickness is around 700 nm.
It is not easy to select the optimum annealing temperature, as several factors are at play. A study involving a series of 480 nm-thick AlN films, grown at a sputtering pressure of 0.05 Pa and temperatures ranging from 1650 °C to 1775 °C, revealed that higher temperatures had no impact on the width of the X-ray diffraction peak in the (0002) direction. However, for the (1012) direction, the peak’s full-width at half maximum fell fr om over 170 arcsec to below 125 arcsec. Note, though, that this gain comes at the price of an increase in macroscopic defects. To minimise these imperfections, an alternative to dialling back the annealing temperature is to turn to a thicker film.
The benefits of a thicker film are quantified by plan-view scanning transmission electron microscopy images of a portfolio of sputtered films annealed at 1700 °C. For these samples, capped with an AlN layer between 0.8-2.8 μm thick that’s been added by MOCVD, threading dislocation density falls from 1.1 x 109 cm-2 to 3.6 x 108 cm-2 when the thickness of the sputtered film is increased from 153 nm to 481 nm.
To drive down the level of threading dislocations even further, Miyake and co-workers have pursued a double sputtering and annealing technique – that is, sputter and anneal, followed by a second sputter and anneal. It brings success, with the threading dislocation density falling to 4.3 x 107 cm-2, according to plan-view transmission electron microscopy.
The team has also compared the characteristics of AlN films grown directly on sapphire by MOCVD, and those added by MOCVD to AlN-on-sapphire templates formed by sputtering and annealing. The latter leads to a far lower screw and mixed dislocation density – it is 2 x 106 cm-2, rather than 3 x 108 cm-2.
Figure 1. As the wavelength of the UV decreases, there is a tendency for
the external quantum efficiency to fall. This plot is provided by
Michael Kneissl from TU Berlin. It is an updated version of Figure 2
from the paper M. Kneissl et al. Nature Photonics 13 233 (2019)
While this lower density may appear a blessing at first glance, it brings its own problems. This is evident when comparing Nomarski microscope images of 1500 nm-thick films of Al0.74Ga0.26N grown by MOCVD on top of both structures. A relatively smooth surface results when this alloy is deposited on MOCVD-grown AlN-on-sapphire, but when it is grown on a template of sputtered and annealed AlN that has a layer of MOCVD grown AlN, the AlGaN is riddled with huge hillocks.
Miyake and co-workers have found that the origin of these hillocks is the spiral growth in the sputtered AlN templates. Due to the low density of screw and mixed-type dislocations, hillocks grow without interruption to a large size. In stark contrast, when there are many more screw and mixed type dislocations – this is the case in the epistructure featuring AlN growth on sapphire by MOCVD – there is interference associated with dislocation-induced spiral growth that creates a flatter surface (as illustrated in Figure 2).
Kenjiro Uesugi, one of Miyake’s colleagues at Mie University, also gave a talk at Photonics West, which included a solution to the hillock issue. Uesugi presented images showing how the density of hillocks varies with an increase in the off-cut angle up to 1°, and remarked: “Increasing the substrate off-cut is quite effective for eliminating the hillock structures.”
To assess the impact of the offcut angle on the quality of the active region, the team from Mie University carried out a cathodoluminescence study on samples featuring quantum wells. Increasing the off-cut angle to 0.6° led to a more intense, narrower photoluminescence peak. But further increases in off-cut angle were not beneficial, resulting in a lower intensity of emission. “We speculate that this behaviour is owing to surface step bunching, and results in degradation of the multi-quantum wells,” argued Uesugi.
The researchers have considered various options for combatting the hillocks. Increasing the temperature eradicates them, but introduces point defects. A better option is to combine a lower growth rate with a high ammonia partial pressure, a step that suppresses excessive surface migration of gallium atoms. Drawing on all these tricks, the team have produced deep-UV LEDs on sapphire with a 0.6° offcut. MOCVD-grown layers were added in a Taiyo Nippon Sanso reactor, using a growth temperature of 1300 °C for the first AlN layer, before dropping the temperature to 1150 °C to grow the device layers.
On-wafer measurements on circular, un-encapsulated 264 nm devices with 160 μm diameter revealed a maximum external quantum efficiency of 1.73 percent. When driven at 20 mA, output is 1.61 mW. In comparison, the control, made on MOCVD-grown AlN, has a maximum external quantum efficiency of just over 1.4 percent.
Ultrathin wells
It is well known that the internal quantum efficiency of the active region of an LED is governed by the interplay between non-radiative and radiative processes. As the emission wavelength heads deeper into the UV, there is a decrease in the photoluminescence lifetime, which is the reciprocal of the non-radiative lifetime. This implies that non-radiative recombination increases at shorter wavelengths.
Working at Kyoto University, Japan, Mitsuru Funato and co-workers have measured the photoluminescence lifetime of quantum-well samples emitting at a range of wavelengths, formed on both sapphire and bulk AlN. Funato told delegates at Photonics West that despite these two classes of sample having markedly different threading defect densities, lifetimes are similar. This led him to believe that the major non-radiative recombination centres are point defects, rather than threading dislocations.
Many years ago, theorists calculated the formation energy for point defects in GaN and AlN. Based on these works, Funato is suggesting that the weakness in the AlGaN quantum wells is the point defect associated with aluminium. He is advocating the use of gallium-rich AlGaN quantum wells. There is much merit to this approach: the optical anisotropy in AlGaN hampers surface emission, which is strong in GaN, aiding light extraction efficiency.
To evaluate this conjecture, Funato and co-workers began by calculating the electron and hole wavefunctions in 1 nm-thick GaN quantum wells and 2 nm-thick AlGaN quantum wells, both sandwiched between AlN barriers and emitting at around 230 nm. The thinner wells had an electron-hole overlap of 0.7, compared with just 0.2 for the thicker variant.
Encouraged by this result, the researchers have gone on to grow ultra-thin GaN quantum wells. They are not the first to do this, but previous work has focused on an MBE-based approach. The team from Kyoto use MOCVD, realising very thin GaN quantum wells with a self-limiting process. Funato told the delegates at Photonics West that he thinks that the first monolayer of GaN stabilises on AlN, but when a second monolayer is added, it evaporates. The upshot is that the thickness of the quantum well is limited to a monolayer, thanks to a bond strength for GaN-on-AlN exceeding that for GaN-on-GaN. Evidence for such a thin well has come from a transmission electron microscopy cross-sectional high-resolution image that show a single monolayer of GaN sandwiched between AlN.
Photoluminescence measurements at a range of temperatures up to 300K have produced promising results. Temperature quenching is less suppressed in the GaN quantum well emitting at 300 nm than its thicker AlGaN cousin, implying a higher internal quantum efficiency. A room-temperature value of 5 percent is estimated for the GaN well, compared with 0.1 percent for the AlGaN variant.
Turning to time-resolved photoluminescence at room temperature has provided additional insight into the nature of the wells. The photoluminescence lifetime is 40 ps in the 1.5 nm-thick AlGaN quantum well, while it is 100 ps in the single monolayer GaN well, indicating that the latter is better at supressing non-radiative processes.
Funato and colleagues have also carried out time-resolved photoluminescence at cryogenic temperatures. Armed with these additional measurements, they have deduced radiative and non-radiative lifetimes. In AlGaN both lifetimes are 40 ps, while in GaN the non-radiative lifetime is 105 ps and the radiative lifetime just 2 ns, due to larger electron-hole overlap.
The team has investigated the emission’s polarisation state by exciting the sample head-on with a 193 nm laser and measuring emission in-plane with a CCD. The researchers found that the transverse magnetic polarisation is slightly stronger than the transverse electric form in the AlGaN quantum well, while the transverse electric dominates in the GaN quantum well, aiding light extraction.
To try and reach even higher values of internal quantum efficiency for ultra-thin GaN quantum wells, the team have switched to the semi-polar plane. A two monolayer-thick GaN quantum well formed on r-plane of sapphire and emitting at around 250 nm produced an internal quantum efficiency of 50 percent.
Deep UV dots
Another option for producing a deep-UV active region, outlined in a presentation by Julien Brault from CNRS-CRHEA in France, is to use AlGaN quantum dots. As these nanostructures provide a high level of spatial confinement for electrons and holes, there is the promise of realisation of a high quantum efficiency in samples with a relatively high defect density.
Working with reearchers at the University of Montpellier and Riber, Brault and his colleagues have produced and studied quantum dot samples that are grown by MBE using the Stranski-Krastanov growth mode. One of the primary goals of this research is to determine the growth condition for realising dots with high internal quantum efficiency.
UV lamps are widely used for water purification, but as UV LEDs improve, they will take an increasing share of this market.
This collaboration forms its dots on sapphire substrates coated with an AlN layer typically 1 μm thick. This template has a dislocation density of the order of 5 x 1010 cm-2. AlGaN dots with an aluminium component of 10-40 percent are grown on Al0.7Ga0.3N, which is also used to clad them. Cross-sectional transmission electron microscopy of these dots, which have a density in the range 3-6 x 1011 cm-2, reveals that the nanostructures are between 1.5 nm and 3 nm high, and have a diameter of around 10 nm.
Brault shared atomic force microscopy images showing that post-growth annealing, at temperatures greater than 800 °C, induces a shift in the shape of the quantum dots from elongated structures to symmetric entities. The change in morphology has no impact on the emission wavelength, implying no change in AlGaN composition. However, there is a tremendous ramp in room-temperature photoluminescence efficiency from 1-3 percent to 10-30 percent, attributed to a significant increase in carrier confinement.
The researchers used time-resolved photoluminescence to investigate the internal quantum efficiency of dots with aluminium components between 10 percent and 40 percent – they span the spectral range 340 nm to 275 nm. The team found that as the wavelength gets shorter, the internal quantum efficiency increases, to around 20 percent in the UVC.
Building on this work, Brault and colleagues gone on to produce LEDs that feature these nanostructures. The devices, sporting a relatively standard structure, have three-to-five quantum dot planes and a p-type GaN contact layer. The latter improves hole concentrations, but increases absorption in the UV range.
The French collaboration has fabricated UVA LEDs with Al0.1Ga0.9N quantum dots, UVB siblings with Al0.2Ga0.8N quantum dots, and expects to reach between 265 nm and 280 nm by increasing the aluminium component to 0.3 to 0.4.
Behaviour of these emitters is quite unlike that of a conventional LED, with performance highly current dependent. When carriers start to flow through the device, initially there is a dramatic decrease in emission wavelength, alongside a reduction in the width of the emission peak. Both trends are believed to stem from fluctuation in the composition and size of the dots, and a screening effect. Further increases in current lead to a second regime, where the emission wavelength stabilises and its peak narrows. It is thought that these characteristics are associated with the injection of carriers into Al0.2Ga0.8N quantum dots. Crank the current up even more and there is an increase in emission wavelength, due to thermal effects, and a reduction in injection efficiency.
These quantum-dot devices have an operating voltage of 10-20 V. External quantum efficiency peaks at just below 0.1 percent, with heating causing this figure to fall.
“The next step will be to fabricate tunnel-junction-based LEDs, where we can expect a strong improvement in the internal efficiency and the extraction efficiency, by avoiding the use of a p-type GaN contact layer,” said Brault.
Figure 2. The low density of screw and mixed-type dislocations in
high-quality AlN of sapphire templates are behind the large hillocks
that form during MOCVD growth. When more screw and mixed-type
dislocations are present, they interrupt the formation of hillocks,
reducing their size. This graphic has provided by Hideto Miyake from Mie
University. More details are given in the following papers: H. Miyake
et al. J. Cryst. Growth 456 155 (2016); S. Xiao et al. J. Cryst. Growth
502 41 (2018); K. Uesegi et al. Appl. Phys. Express 12 065501 (2019).
Extracting emission
One of the biggest weaknesses with a conventional deep-UV LED is its low extraction efficiency. Hideki Hirayama from Riken University, Japan, highlighted this impediment in his talk, before discussing a variety of architectures that can address this issue.
According to Hirayama, for a standard UVC LED, extraction efficiency can be as low as just 4-8 percent, due to a p-GaN contact that absorbs light and internal reflections in the sapphire substrate. He explained that one option for getting more light out of the chip is to add a photonic crystal structure on the n-side of the device, but this only increases the extraction efficiency to 12-15 percent. A far better strategy, he said, is to address the weaknesses of the p-side, by switching to a highly reflective electrode and a transparent contact layer. Combine this with a pillar-based technology on the n-side of the device – claimed to be a better option than a photonic crystal structure for boosting extraction from the n-side – and light extraction efficiency could exceed 70 percent.
Hirayama and co-workers produce their UVC LEDs on sapphire, beginning with the growth of a five-step multilayer buffer, created by ammonia pulse flow growth. This 3.8 μm-thick structure, which has a dislocation density of 5 x 108 cm-2, has provided the foundation for producing a portfolio of UVA-UVC LEDs. Devices emitting at 270 nm produce an output of more than 50 mW. Internal quantum efficiency is estimated to be 54 percent, according to photoluminescence measurements made at a variety of temperatures. The team has devoted several years to developing approaches to increasing the light extraction efficiency of deep-UV LEDs. Back in 2017, these researchers reported results for devices featuring: transparent p-AlGaN layers, a reflective p-type electrode, an AlN buffer on patterned sapphire, and a lens-like resin mold. This powerful combination yielded a world-record light-extraction efficiency of 20.3 percent for a 275 nm LED, according to Hirayama.
While this is impressive, Hirayama is quick to point out that more work is needed, given that the wall-plug efficiency is only around 6 percent. Losses can be attributed to the use of p-AlGaN, which leads to a hike in the operating voltage – it increases from around 5.5 V to 9.1 V. Recently, the team evaluated the benefits of inserting a photonic crystal reflector in the p-side of the LED. Simulations suggest a three-fold enhancement in light-extraction efficiency – this could hit 54 percent, when pairing this novel reflector with p-type AlGaN.
Hirayama and co-workers have produced photonic crystal UV LEDs by nano-imprinting and dry etching. These devices, containing an array of holes with a 160 nm diameter and depth of 338 nm in the p-side, alongside a highly reflective mirror made from the combination of nickel and magnesium, operate with an external quantum efficiency of up to 10 percent.
To reduce the operating voltage, the team has also produced variants with p-type GaN. Introducing the photonic crystal is beneficial, as it doesn’t alter current-voltage characteristics, but enhances light extraction by 70 percent. That’s not as big a gain as promised by simulations, because the photonic crystal is yet to be optimised. The latest generation of UVC devices produce between 30 mW and 50 mW.
Even higher powers are produced by large flip-chip structures. For devices 1.2 mm by 1.2 mm in size and driven at 2 A, output is as high as 140 mW.