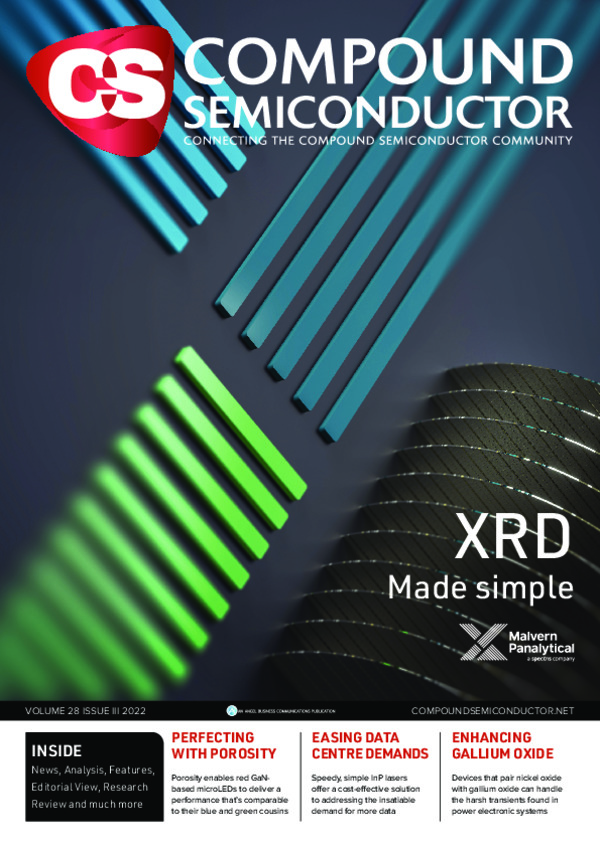
Easing data-centre demands with directly modulated lasers
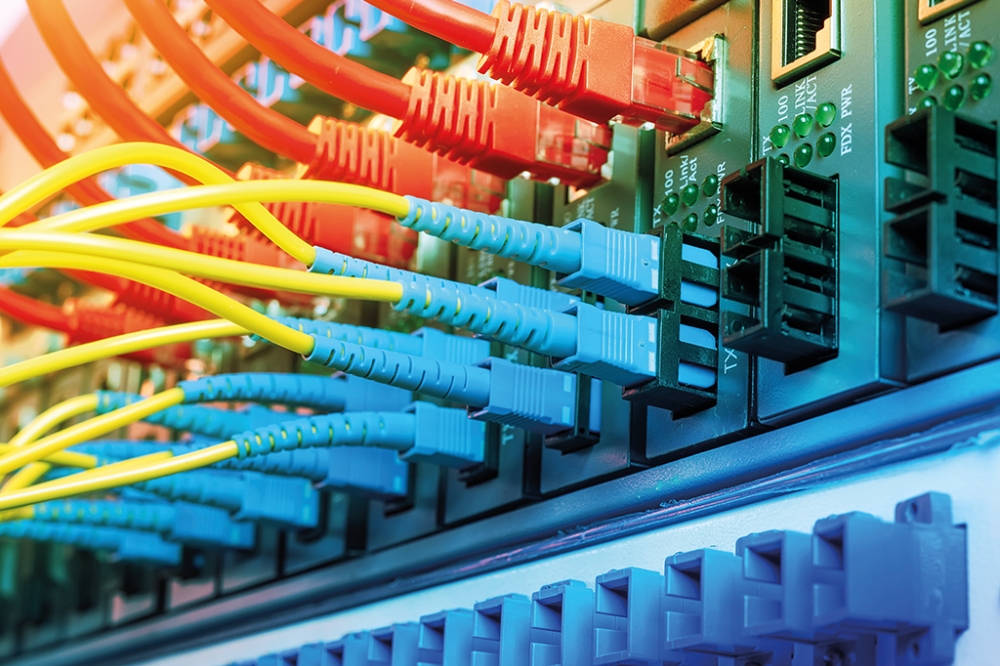
Simple InP lasers operating at 100 Gbit/s will provide a cost-effective
solution to addressing the insatiable demand for more data
BY
MASARU ONGA, TAKAYUKI NAKAJIMA, YUJI SEKINO, AKIRA NAKANISHI, NORIKO
SASADA, RYOSUKE NAKAJIMA, HIRONORI SAKAMOTO AND KAZUHIKO NAOE FROM
LUMENTUM JAPAN
To meet the continued explosion of network traffic there has to be an expansion in capacity. Part of the solution is to introduce new components. They must cost no more than their predecessors, to prevent the budget from spiralling out of control; and they must minimise power consumption to keep down running costs and restrict the carbon footprint of optical infrastructure.
A particularly significant proportion of global network capacity is associated with the data traffic in data centres and 5G wireless networks. In response to a recent increase in demand, over the last few years designers of data centres have moved on from deploying optical transceivers for 100GbE, which can for example be formed from four lasers running at 26 Gbit/s, to 400GbE, a data rate that may be realized with four 106 Gbit/s lasers. For these 106 Gbit/s sources, 53-GBaud four-level pulse amplitude modulation (PAM4) has been widely adopted, enabling a transmission speed of 106 Gbit/s. PAM4 is expected to be utilized in optical transceivers in data centres, for example, in 400GBASE-DR4 or 400GBASE-FR4 in 400GbE. In addition, 106 Gbit/s PAM4 technology is being considered for 800GbE short-reach applications, such as 800G-PSM8, formed from eight lasers operating at 106 Gbit/s.
Figure 1. (a) The structure of a ridge-waveguide directly modulated laser. (b) Cross-sectional view
One very promising candidate for providing 106 Gbit/s lasers in these multi-wavelength transceivers is the directly modulated distributed-feedback (DFB) laser. Compared with alternatives, such as the electro-absorption modulator integrated DFB laser, it has many advantages, including a simple structure, a low driving current, a small size and cost effectiveness. What’s more, the directly modulated DFB laser is advantageous from a thermal management perspective. In the preceding 100GbE era, this class of laser operated without thermoelectric cooler control over a wide temperature range – and the anticipation is that at 400GbE and beyond, it will continue to operate in uncooled setups while drawing very little power. Also, developers of directly modulated lasers for this application must consider the lasing wavelengths that will be required. For example, for the coarse wavelength-division multiplexing (CWDM) range for 400 GbE, four sources must span 1271 nm to 1331 nm and each emit at wavelengths slightly offset from one another.
Options for these applications include a variety of directly modulated InP-based laser architectures. Candidates include the distributed-reflector laser with a buried heterostructure, and variants with a ridge-shaped buried heterostructure and a p-n blocking buried heterostructure. However, all these designs are held back by the use of some additional structures like a buried heterostructure – it requires additional epitaxial growth that adds complexity and cost.
Offering far more promise is the ridge-waveguide directly modulated laser. With the potential to provide a simple, easy-to-fabricate structures for uncooled 106 Gbit/s operation, it is an excellent candidate for ensuring high-volume production of cost-effective transceivers. However, success is not trivial, with innovation required to ensure an increase in bandwidth. Introducing external optical feedback can accomplish this, but it additionally requires external structures, so is not preferable.
Working with this restraint, our team from Lumentum, Japan, has developed ridge-waveguide directly modulated lasers operating at four different wavelengths within the CWDM range (see Figure 1(a)). To achieve a high bandwidth that enables 106 Gbit/s operation, our lasers exhibit a high relaxation oscillation frequency, even at elevated temperatures, thanks to their high differential gain and high optical-confinement factor. Drawing on these strengths, our transmitters are capable of producing clear 106 Gbit/s PAM4 eye openings from 25 °C to 85 °C.
Figure 2. (a) Light-current characteristics, (b) spectra and (c) fr
(relaxation oscillation frequency)-slope characteristics of four
directly modulated lasers
We have also evaluated the transmission capabilities and the bit-error rate characteristics of these sources, considering links of 500 m and 2 km. Strong results from these investigations showcase the great potential of our lasers for making cost-effective transceivers with low power consumption and high data-transmission rates.
Figure 3. 106 Gbit/s PAM4 optical eye-diagrams of the four directly
modulated lasers at 25 °C, 50 °C and 85 °C with drive currents of 50 mA,
60 mA and 85 mA, respectively. 106 Gbit/s PAM4 eye diagrams were
evaluated by using a Keysight M8045A pattern generator to produce an
electrical signal with a short-stress pattern, random quaternary test
pattern. The directly modulated lasers were modulated at up to 35 mA
peak-to-peak with a single-ended signal inputted through an RF
amplifier. DC bias (Ib) was also applied via a bias tee just before an
RF probe. Optical waveforms were detected by a Keysight N1092C sampling
oscilloscope. The eye diagrams were measured with a 5-tap feed-forward
equalizer in a back-to-back configuration. The outer extinction ratio,
adjusted to 3.0 dB, was limited by a signal-voltage of the pattern
generator output and the RF amplifier gain.
Speedy designs
Our latest devices draw on our previous lasers, namely the development of ridge-waveguide directly modulated lasers operating at 25 Gbit/s and 50 Gbit/s. Using this foundation, we have developed lasers that: are capable of higher speeds; emit at four different wavelengths; and feature a high differential gain and a high optical confinement factor.
Semiconductor multilayers of our lasers were epitaxially grown on n-type InP substrates by MOCVD. To ensure high-temperature operation, the photoluminescence wavelength for each lane targeted optimal wavelength detuning. By optimising the band structure of the InGaAlAs multi-quantum-well layers and the zinc doping, we realised a high differential gain, even at high temperatures. Contributing to optical confinement were the multi-quantum-well layers and the separate-confinement heterostructure layers – working together, they increased interaction between the electron and photon systems.
We devoted much thought to the design of our ridge-waveguide lasers. For all four lanes we have employed a cavity length of 150 µm and normalized coupling coefficients of around 2.0. We suppressed longitudinal spatial hole-burning with an asymmetric corrugation-pitch modulated grating. The grating pitches for our four directly modulated lasers were set to 195 nm, 198 nm, 202 nm and 205 nm, corresponding to the CWDM range, namely, 1271 nm (Lane 0), 1291 nm (Lane 1), 1311 nm (Lane 2) and 1331 nm (Lane 3). To complete device fabrication, we added a p-electrode pad on a buried organic insulator (this decreased parasitic capacitance and ultimately prevented high-frequency performance degradation); and we deposited anti-reflection and high-reflection coatings on the front and rear facets of our lasers.
Figure 4. 106 Gbit/s PAM4 eye-diagrams of the four directly modulated
lasers in a back-to-back configuration and after 500 m and 2 km
transmission at 85 °C and a drive current of 85 mA.
To evaluate our devices, we mounted four directly modulated laser chips on ceramic carriers with resistors that ensured 50 Ω impedance matching. We used this chip-on-carrier configuration, alongside a thermoelectric cooler that controlled the device temperature, to gain insights into all characteristics of our laser chips – including electro-optical responses, waveforms and bit-error rates.
Our plots of light output at a range of currents and temperatures (see Figure 2 (a)) showed that at 80 °C threshold currents range from 13.7 mA to 16.3 mA. When biased at 70 mA, our laser output exceeded 6.7 mW, a value sufficient for optical networks. Spectral measurements showed that all four lasing wavelengths were well separated into each lane. Driven at 50 mA at 25 °C, all four directly modulated lasers had a side-mode suppression ratio of over 45 dB, indicating stable single-mode lasing in spite of the high normalized coupling coefficients.
Measurements of the electro-optical response revealed values of the relaxation oscillation frequency between 3.8 and 4.4 at 25 °C, and 2.8 and 3.0 at 85 °C, indicating a high bandwidth for all four directly modulated lasers. Driven at 70 mA and operating at 85 °C, 3 dB bandwidths for all four lasers were 20.1 GHz.
To 100 Gbit/s
Measurements on our lasers at device temperatures from 25 °C to 85 °C revealed eye openings over four levels using a back-to-back geometry (see Figure 3). In this configuration, measurements on the four directly modulated lasers produced values for a metric known as the Transmitter and Dispersion Eye Closure Quaternary (TDECQ) of 2.6 to 3.0 dB at 25°C, and 3.1 to 3.4 dB at 50  °C. These values satisfy the 400GBASE-FR4 specification of 3.4 dB.
Using single-mode fibre with zero dispersion wavelength at 1310 nm, we evaluated the transmission properties of our directly modulated lasers from 25 °C to 85 °C. We aimed to check the feasibility for the 400GBASE-DR4 specification for 500 m transmission, and 400GBASE-FR4 for 2 km transmission. Clear eye openings indicate successful transmission even at 85 °C (see Figure 4).
Figure 5. Transmitter and Dispersion Eye Closure Quaternary versus
transmission distance in four directly modulated lasers at 25 °C.
Note, though, that we found that chromatic dispersion can distort the eye diagram during transmission, especially in Lanes 0 and 3, which are far from the zero-dispersion wavelength of the single-mode fibre. So, to clarify the effect of chromatic dispersion, we plotted TDECQ values in a back-to-back configuration, and for transmission over distances of both 500 m and 2 km at 25 °C. These measurements showed that TDECQ values for Lane 0 and 1 gradually decrease as waveforms are transmitted, due to negative dispersion; while those for Lane 2 and 3 slightly increased, owing to positive dispersion. These findings are consistent with the relationship between each lasing spectrum and the zero-dispersion wavelength of the single-mode fibres.
Figure. 6. Bit-error rate characteristics of Lane 2 in a back-to-back
configuration and after 500 m of transmission at device temperatures of
25 °C, 50 °C, 70 °C and 85 °C. A gray-corded optical signal with a
pseudo-random binary sequence (PRBS) 231−1 pattern was received by a
bit-error-rate tester consisting of a commercially available QSFP28
transceiver compatible with 100GBASE-FR1. An optical attenuator
controlled optical power received at the transceiver.
For both a back-to-back configuration and transmission through 500 m of fibre, we measured bit error rates at various values for the optical modulated amplitude for Lane 2, for laser temperatures of 25 °C, 50 °C, 70 °C and 85 °C (see Figure 6). Signals over optical modulation amplitudes of -3.5 dBm were not detected, due to losses related to fibre transmission and switching. While bit error rates increased with optical modulation amplitude as the temperature of the laser rose, it is still possible to realise excellent bit error rates that are below the threshold of KP4-FEC in 400GbE – that is, below 2.2 × 10−4 – at up to 85 °C. These characteristics for the bit error rate directly demonstrate the feasibility of our uncooled directly modulated lasers for 400bE applications.
Our extensive measurements highlight the capability of our directly modulated lasers for serving in optical networks. These ridge-waveguide devices deliver 106 Gbit/s operation at up to 85 °C within the 1.3 µm CWDM range, with measurements of eye openings underscoring the capability for data delivery over several kilometres of fibre. Thanks to the simple design and fabrication process, allied to low power consumption and lack of need for cooling, our lasers are a very promising candidate for providing cost-effective transceivers for hyperscale data centres.
Further reading
N. Sasada et al. J. Lightwave Technol. 37 1686 (2019)
T. Nakajima et al. J. Lightwave Technol. 40 1815 (2022)