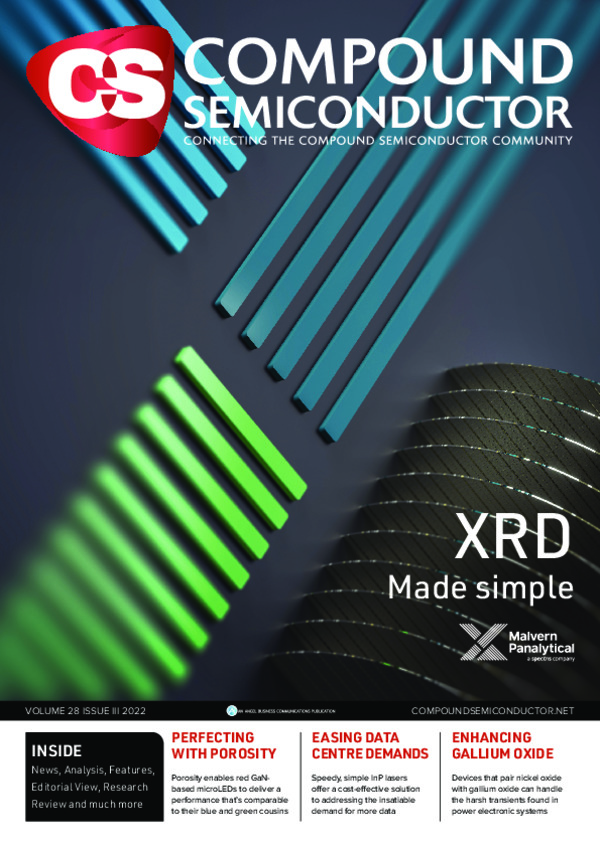
NiO equips Ga2O3 with bipolar conduction
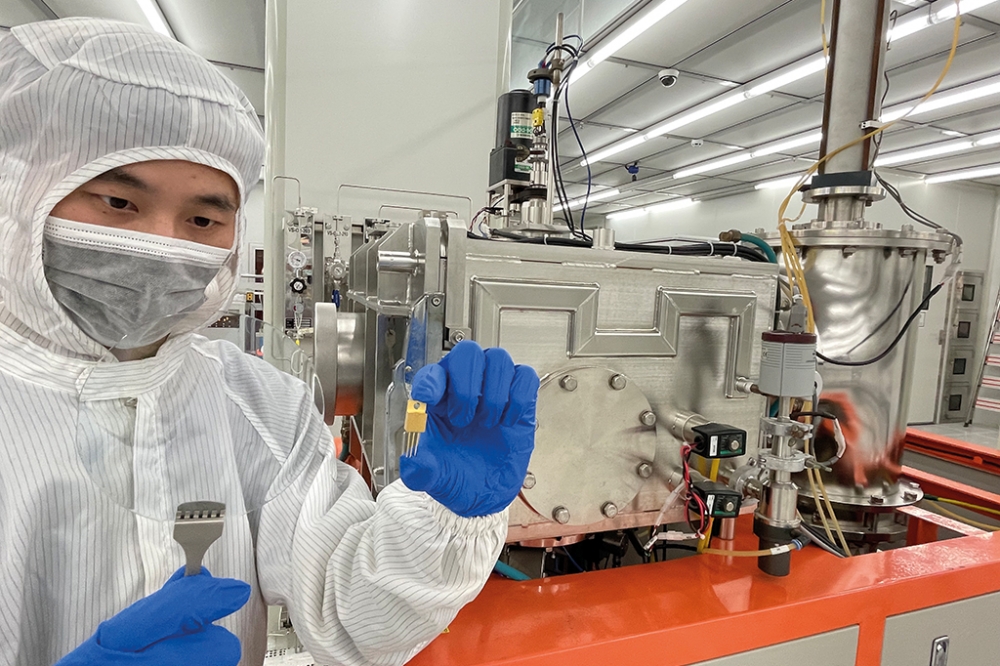
Diodes formed from p-type NiO and n-type β-Ga2O3 embrace the harsh transients found in power electronic systems
BY JIANDONG YE, HAI LU AND RONG ZHANG FROM NANJING UNIVERSITY
βTHERE’S NO DOUBT that power electronic circuits are playing an ever-increasing role in our lives. They are key building blocks in big-data centres, electric vehicles, cloud computing and the internet of things. We have a diverse network of power electronic equipment to thank for keeping us immersed in the pleasure of technology at all times. To provide this level of service, circuits must operate for a long time without failure and handle unavoidable high-voltage/current transients.
These transients – momentary spikes in voltage or current – tend to be accompanied with lightning, electrostatic discharge. When power circuits experience such a sudden change in the switching process caused by transients, this threatens to disrupt or damage power devices connected to signal or power lines (see Figure 1). Such transients can deteriorate power systems, because power electronic devices have to operate under critical conditions of high voltages and large currents. Consequently, there is tremendous desire to increase the robustness of power devices against transients, as this will ensure the availability of essential building blocks for power modules providing safe operation.
Figure 1. The NiO/β-Ga2O3 power rectifiers, with different bevel angles
adjusted by a magnetron sputtering system. Inset: schematic
cross-section of NiO/β-Ga2O3 heterojunction diodes and a Ni/β-Ga2O3
Schottky barrier diode. The transient energy shocks that degrade device
performance stem from the lumped or parasitic elements in the power
circuit.
One option for ushering in an era of high-reliability power circuits is to replace silicon devices with those made from wide-bandgap semiconductors, which are well-suited to delivering high-temperature, high-power operation. Amongst these wide bandgap semiconductors, β-Ga2O3 has many attributes: an ultra-wide bandgap, an ultra-high breakdown electric field, and a decent electron mobility. These virtues make this oxide a very competitive candidate for high-power applications. Furthermore, Ga2O3 is renowned for its high thermal stability, ensuring high-temperature operation; and for its short minority carrier lifetime, enabling fast switching with low power losses.
Figure 2. Top-view microscopy image of the heterojunction barrier
Schottky diodes with p-NiO ring width/spacing of (a) 2 µm and (b) 3 µm.
(c) NiO/β-Ga2O3 Schottky barrier diodes with a uniform field limiting
rings (FLRs) with a width/spacing of 2 µm. (d) and (e) Atomic force
microscopic images of (a). (f) Height profile of p-NiO rings extracted
from (e).
Unfortunately, the potential of β-Ga2O3 is yet to be unleashed. The biggest sticking point is the absence of p-type conduction, which obstructs the design of bipolar power devices. Due to this, the majority of reported β-Ga2O3 power rectifiers detail the design and capability of regularly unipolar Schottky barrier diodes. With these devices, it is very hard to realise high-voltage ratings and high-power levels, due to the limited barrier height. What’s more, this form of diode is particularly susceptible to self-heating at high current levels. So today, despite much effort, unipolar Schottky barrier diodes are restricted to serving in the terminal designed domain, primarily because this accommodates premature breakdown and transient overvoltage instabilities.
Figure 3. (a) Comparison of breakdown characteristics for double-layer
NiO films (S1) and single-layer NiO films (S2). (b) Forward
current-voltage characteristics of devices S1 and S2. (c) Pulsed
current-voltage (I–V) characteristics and corresponding on-resistance
(Ron) of the 1 mm2/ 3 mm2 heterojunction diodes (HJDs). (d) Reverse I-V
characteristics. (e) Ron versus blocking voltage (BV) benchmarks of the
bevelled-mesa HJDs against the 1 mm2 Ga2O3 Schottky barrier diodes
(SBDs)/junction barrier Schottky diodes (JBS)/ HJDs, GaN SBDs, and
commercial SiC SBD.
A solution to breaking this deadlock is to introduce power rectifiers with bipolar conduction – this will banish the trade-off between the reverse blocking capability and the forward current output. Implementing p-n bipolar structures in power devices will open the door to simple device fabrication processes and enhanced reserve-blocking voltages, while delivering extremely high current densities with reduced on-state resistances. Merits of such a strategy include a large safe-operation-area under both a forward surge-current and reverse transient-energy spikes, as well as the potential for fast switching.
Aiming to make this happen is our team led by Rong Zhang from Nanjing University. We kickstarted our efforts at constructing β-Ga2O3 p-n bipolar devices by carefully considering several foreign p-type semiconducting oxide materials that promised to overcome the technological bottleneck of p-type β-Ga2O3. Piquing our interest during the search through the p-type transition metal oxide family came NiO. It is an attractive, well known transparent hole-transport layer in solar cells and photodetectors, thanks to its decent hole conductivity and its desirable transparency that stretches from the ultraviolet to the near infrared. It is believed that the origin of this oxide’s high hole concentration is the formation of Ni3+ from Ni2+ nearby nickel vacancies, coming from the reaction with excess oxygen atoms. This chain of events creates holes that are localized on the nickel sites and offer the acceptable mobility.
Thanks to this great set of attributes, NiO is our material-of-choice for constructing p-n heterojunctions with β-Ga2O3. Back in 2020, we reported the first demonstration of a double-layered p-NiO/n-Ga2O3 heterojunction power rectifier, which had a breakdown voltage of up to 1.86 kV. Since then we have devoted much effort to building on this triumph, and advancing β-Ga2O3 based bipolar power devices. Recent successes include: an enhanced reverse blocking capability; high forward current outputs, together with nanosecond reverse recovery; a high power-conversion efficiency; and more than a million-time overvoltage surge-current ruggedness.
Building heterojunctions
Using our in-house advanced material growth and microfabrication facilities, we have produced a portfolio of NiO/β-Ga2O3 vertical heterojunction diodes on the conductive, tin-doped (001) β-Ga2O3 substrate. To make these devices, we developed room-temperature, selective-area growth of NiO. Using radio frequency (RF) magnetron sputtering, a technique used in industry, and have heteroepitaxially grown high-quality, single-crystalline NiO thin films on c-plane sapphire, ZnO and GaN substrates. We have found that the crystalline quality of NiO is sensitive to the exposed strain and to the in-plane crystallographic symmetry of the substrates. For instance, NiO epilayers have high-quality single crystallinity on (-201) β-Ga2O3 substrates, but exhibit polycrystalline features on the (001)-oriented β-Ga2O3. There is also a concern that the sputtering process could introduce plasma damage to the NiO/β-Ga2O3 interface.
To maximize the advantages of NiO technology in device fabrication, those within our team that are led by Jiandong Ye have developed relatively mild sputtering conditions for the growth of this oxide, and equipment that allows small adjustments to the deposition angle. Efforts have also been directed at developing optimized thermal treatment to improve the interface quality of NiO/β-Ga2O3 heterostructures. Introducing these well-developed processing recipes has enabled us to fabricate high-performance NiO/β-Ga2O3 heterojunction diodes with a low interfacial state density.
Another tremendous advantage of our sputtering technique, which stems from its adjustability and flexibility, is that it is possible to naturally form devices with a bevelled mesa termination structure – this avoids any dry etching damage, and results in an architecture that greatly suppresses the peak electric field. The use of this non-invasive formation of termination, rather than dry etching, has previously been employed to realize heterojunctions with other n-type wide bandgap semiconductors, such as those in the AlGaN/GaN system, where much effort has focused on developing normally-off transistors. By turning to sputtering, we are using a film growth technique that offers compatibility between our Ga2O3-based power devices and the mature CMOS platforms.
Drawing on our readily available NiO/β-Ga2O3 p-n heterojunction, it has been relatively easy for us to pursue several strategies for electric field engineering. We have fabricated a series of high-performance power devices, including β-Ga2O3 heterojunction barrier Schottky diodes, heterojunction FETs, reduced-surface-field MOSFETs and the first β-Ga2O3-based lateral superjunction MOSFETs. With the heterojunction barrier Schottky diode, where the NiO/β-Ga2O3 p-n heterojunction serves as field plates and field limiting rings, we can freely modulate the electrical parameters of this power rectifier that combines a low on-state conduction loss with a superior reverse-blocking capability (see Figure 2).
Intriguing electrostatic performances
Electrical measurements on a range of our power rectifiers reveal the impact of the hole concentration in p-NiO on the voltage blocking capability. When we reduced the hole concentration from 3.6 × 1019 cm-3 to 5.1 × 1017 cm-3, this lifted the breakdown voltage from 0.94 kV to 1.86 kV (see Figure 3 (a)). Unfortunately, the far lighter doping had unwanted consequences, increasing the differential specific on-resistance of the NiO/β-Ga2O3 heterojunction diode and exacerbating on-state power loss. Due to this, we have to limit the forward current output for this device to 150 A/cm2 (see Figure 3 (b)).
By employing more elaborated techniques and expanding device dimensions, we have realised high current outputs. To this end, we have fabricated large-scaled NiO/β-Ga2O3 p-n heterojunction diodes with sizes of 1 mm2 and 9 mm2. These power rectifiers deliver forward currents of over 12 A and 70 A for the smaller and larger devices, while on-resistances are just 260 mΩ and 65 mΩ, respectively. The expansion of conduction area does introduce concerns, however, with blocking voltages unevenly distributed, due to severe electric-field crowding at the NiO/β-Ga2O3 mesa edge.
One way to address this challenging issue is to use a bevelled mesa design with different bevel angles. Recently, by implementing a bevel mesa design with a 6° bevel angle, we have boosted the blocking voltage of our NiO/β-Ga2O3 heterojunction diodes from 1.1 kV to over 1.9 kV (see Figure 3 (d)) while maintaining their excellent forward conduction characteristics.
We can benchmark our NiO/β-Ga2O3 heterojunction diodes using Baliga’s power figure-of-merit. For our large-scaled power rectifiers, this benchmark produces a value of over 1.8 GW/cm2. It is a value that approaches the limit of SiC unipolar devices, and is comparable to state-of-the-art large-sized Ga2O3 Schottky barrier diodes rectifiers, as well as commercial Schottky barrier diodes based on GaN and SiC (see Figure 3 (e)).
Figure 4. The team led by Hai Lu at Nanjing University has developed a
high-speed, high-reliability dynamic test system to characterize the
dynamic performance of power devices based on wide-bandgap
semiconductors.
Dynamic switching characteristics
For emerging Ga2O3 power devices, systematic investigations of dynamic characteristics are still lacking. This is a significant omission, because an insight into the dynamic characteristics of these devices is crucial for designers of power electronic circuits for practical applications. To evaluate the dynamic performance of power devices, those within our team that are led by Hai Lu have developed a high-speed, high-reliability dynamic testing system. This is capable of evaluating all switching parameters normally listed in device datasheets (see Figure 4). Measurements undertaken with this set-up include the double-pulse switching test, which features a fast-speed commercial GaN transistor, serving as an active switch that is capable of on/off switching within 50 ns (Figure 5 (a)).
Traces on one of our oscilloscopes show that during the switch-off process, the reverse recovery losses in our NiO/β-Ga2O3 heterojunction diodes are almost zero, while the switching time is around just 12 ns (see Figure 5 (b)). We attribute this fast-speed switching to a sub-nanosecond minority-carrier lifetime, and limited storage charges in the drift region that are easily extracted by the high reverse bias during the switching process.
Figure 5. (a) Schematic of the double-pulse test circuit. The fast-speed
commercial GaN gate-injection transistor has been used as a control
switch. (b) Reverse recovery characteristics of different diodes. (c)
The power-factor-correction (PFC) circuit with the packaged Ga2O3
heterojunction diode. (d) System efficiency verses output power (Pout)
of a 500-W PFC circuit at switching frequencies of 0.1 MHz and 0.5 MHz
and the extracted case temperature of the three test samples at 0.3
MHz/500 W.
A straightforward, valuable way to evaluate power devices is to assess their power-conversion capability. We have taken this approach, constructing a 500 W power-factor-correction circuit to exploit the power-conversion efficiency of our NiO/β-Ga2O3 heterojunction diode (see Figure 5 (c)). In this circuit our device has a power conversion efficiency of 98.5 percent, and provides stable switching for the 100 minutes under test (see Figure 5 (d)). These figures underscore the promise of our NiO/β-Ga2O3 rectifiers for serving in high-power, high-efficiency power applications.
The Achilles’ heel of β-Ga2O3 is its rather low thermal conductivity. This well-known flaw is of such concern that it has led some to question whether Ga2O3 is a viable material for making power devices. However – and this will come as a surprise – in our NiO/β-Ga2O3 power rectifiers the self-heating effect caused by this weakness is not a major worry. When elevating the temperature of our rectifier from 25 °C to 200 °C, we found that our device exhibited an enhanced current capability, alongside a significant reduction in on-resistance. The case temperature for our device at a switching frequency of 0.3 MHz was only just over 60 °C, and more than 10 °C lower than that of the commercial silicon fast-recovery diode. Note that this is not the case for other forms of Ga2O3 device – in those variants, there is significant self-heating that results in a high junction temperature and ultimately severe degradation of the forward-current output.
Enhancing reliability
The rapid progress that has been realised with Ga2O3 power devices has not been accompanied by a systematic characterization of device reliability. Particularly lacking are reports of device behaviour under large forward surge-currents and high reverse transient-energy spikes. When high currents run through the device for a long duration, or it experiences current overshoot/oscillation surging, this can generate a huge amount of Joule heat within the resistive diodes. Heat accumulating inside these diodes leads to an abrupt rise in junction temperature, and in turn a hike in on-resistance that deteriorates device performance.
Using a resonance circuit to generate a half-sinusoidal surge current, we have found that our Ga2O3 heterojunction diode produces a peak surge of 45 A, with a high surge power of 423 W. These values are far higher than those of the
Ga2O3 Schottky barrier diodes counterpart (see Figure 6). The superior conduction capability of our devices comes primarily from the bipolar conductivity modulation effect in a dynamic/transient level, which is induced by minority carrier injection and diffusion.
Figure 6. (a) Surge current-voltage (I-V) waveforms of the 9-mm2
heterojunction diode (HJD) and Schottky barrier diode (SBD). Inset: The
resonance circuit generates a 10 ms half-sine surge current pulse to go
through the device under test. (b) The corresponding surge power and
energy.
In addition to withstanding the released energy stored in the inductors of inductive switching circuits, such as power-factor-correction circuits, in order for power devices to offer good levels of reliability, it is critical that they provide transient overvoltage ruggedness – that is a measure of the dynamic blocking voltage.
To see if this is the case, we have used an unclamped inductive switching test setup to determine the reverse overvoltage reliability of our Ga2O3 rectifiers. We have found that our device with a 6° bevel angle exhibits an ultra-high dynamic blocking voltage of 2.23 kV and can handle a repetitive breakdown over 1 million times (see Figure 7). These results demonstrate that appropriate electric field management can ensure advantageous overvoltage ruggedness. Several strengths of the device are behind its superior breakdown performance. Firstly, there is minimal electric-field-crowding at the small-angle mesa edge, in stark contrast to the large electric field found in the steep-mesa heterojunction diode, containing sharp corners that are the cause of premature breakdown.
Figure 7.(a) The unclamped inductive switching (UIS) current and voltage
waveforms of the bevelled-mesa Ga2O3 heterojunction diode (HJD) and the
step-mesa Ga2O3 HJD, respectively. Inset: schematic of the test
circuit. (b) Repetitive UIS waveforms and parameters.
Furthermore, our latest devices benefit from: an extension of the off-state p-n depletion region that contributes to the suppressed leakage current; and our room-temperature sputtering process, which reduces interfacial traps and trims the leakage current. The benefits are significant – we are breaking new ground when experimentally verifying that NiO/β-Ga2O3 heterojunction diodes are capable of delivering robust reliability, both in forward and reverse operation; and we have overcome the grand challenge of transient surge-current and breakdown in actual power circuits.
Our successes are important breakthroughs, taking this device one step further towards providing the key building block in a new generation of power electronics delivering unprecedented levels of power, efficiency and reliability.
Further Reading
H. H. Gong et al. “1.37 kV/12 A NiO/β-Ga2O3 heterojunction diode with nanosecond reverse recovery and rugged surge-current capability,” IEEE Trans. Power Electron. (2021)
H. H. Gong et al. “A 1.86-kV double-layered NiO/β-Ga2O3 vertical p–n heterojunction diode,” Appl. Phys. Lett. (2020)
F. Zhou et al. “1.95-kV beveled-mesa NiO/β-Ga2O3 heterojunction diode with 98.5% conversion efficiency and over million-times overvoltage ruggedness,” IEEE Trans. Power Electron. (2022)
H. H. Gong et al. “β-Ga2O3 vertical heterojunction barrier Schottky diodes terminated with p-NiO field limiting rings,” Appl. Phys. Lett. (2021)
F. Zhou et al. “Over 1.8 GW/cm2 beveled-mesa NiO/β-Ga2O3 heterojunction diode with 800 V/10 A nanosecond switching capability,” Appl. Phys. Lett. (2021)