A new way to make efficient GaN-based VCSELs
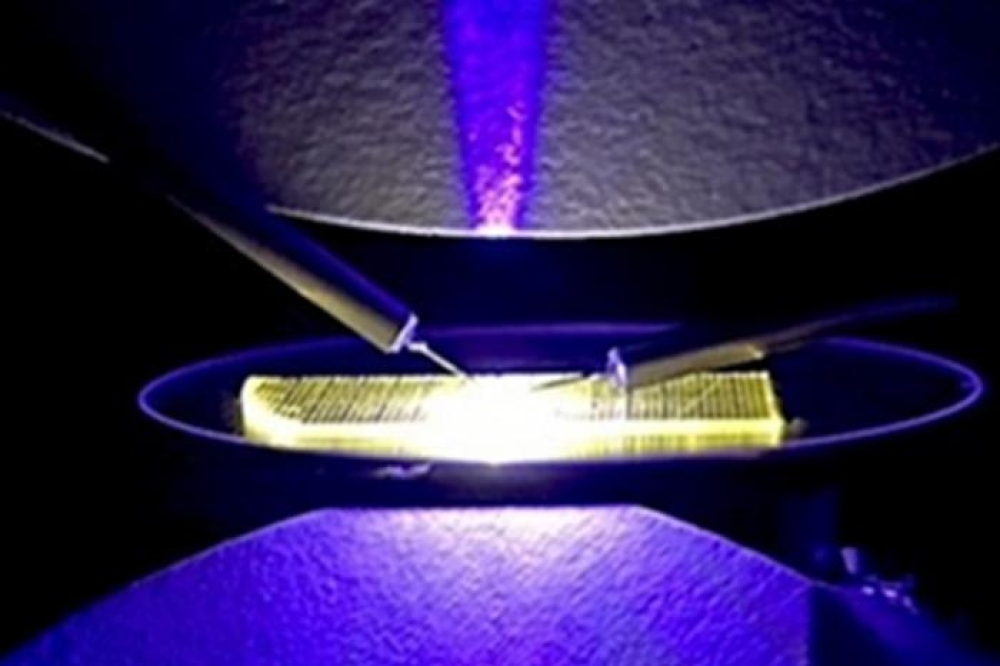
Japanese team develops innovative method for precise cavity length control
GaN-based VCSELs are made of two layers of semiconductor mirrors (distributed Bragg reflectors or DBRs), separated by active GaN-semiconductor layers, which form the optical resonant cavity. The length of this cavity is crucial for controlling the target laser wavelength.
To date, two types of GaN-based VCSEL structures have been developed. One has a bottom dielectric DBR and the other has a bottom AlInN/GaN DBR. Both of them result in VSCELs with over 20 mW of light output power and an over 10 percent wall plug efficiency (WPE).
However, the AlInN/GaN DBR has a narrow stop wavelength bandwidth, which allows light only within a narrow wavelength range to be emitted from the VCSELs. Moreover, the traditional cavity length control method, which involves pre-experiments with test cavity layers for the determination of their growth rate, results in an error between the estimated and final thickness of the VCSEL cavity. This error could lead to resonance wavelengths being out of the narrow stop bandwidth of the AlInN/GaN DBR, significantly affecting performance.
To address this issue, in a recent study, researchers from Japan, led by Tetsuya Takeuchi from the Department of Materials Science and Engineering at Meijo University, developed a new in situ cavity length control method for a GaN-based VCSEL optical cavity.
By precisely controlling the growth of GaN layers using in situ reflectivity spectra measurements, the researchers achieved precise cavity length control with only a 0.5 percent deviation from the target resonance wavelength.
Now, they have further expanded this innovative technique and demonstrated cavity length control of a complete VSCEL.
Takeuchi explains: “The cavity of a VCSEL contains not only GaN layers but also an indium tin oxide (ITO) electrode and a niobium pentoxide (Nb2O5) spacer layer, which cannot be controlled with the same in situ reflectivity spectra measurement system. In this study, we developed a technique to precisely calibrate the thickness of these additional layers, resulting in highly efficient VCSELs.”
The study was published in Volume 124, Issue 13 of the journal Applied Physics Letters on March 28, 2024.
To calibrate the thickness of the additional layers, the researchers first deposited the ITO electrodes and Nb2O5 spacer layers with varying thicknesses on the GaN test structures that were grown using the in situ cavity control. Given that the in situ reflectivity measurement cannot be used for these additional layers, they directly evaluated the resonance wavelength of these test cavity structures using ex situ reflectivity spectra measurement. The obtained resonance wavelengths were redshifted, meaning the wavelengths were increased with an increase in the thickness of ITO and Nb2O5 layers
Next, the researchers mapped the resonance wavelength shifts as a function of the ITO and Nb2O5 layer thicknesses, obtaining accurate information about their optical thicknesses. They used this information to precisely calibrate the thickness of the ITO and Nb2O5 layers towards the target VCSEL resonance wavelength. The deviation of resonance wavelength control resulting from this method was sufficiently low, within 3 percent, comparable to the in situ control method in terms of optical thickness.
Finally, the researchers fabricated GaN-VCSELs with aperture sizes ranging from 5 to 20 µm by implementing the tuned ITO electrodes and Nb2O5 spacer layers into the VCSEL cavity grown using the in situ cavity control. The peak emission wavelength of these VCSELs had a deviation of merely 0.1 percent from the designed resonance wavelength. Notably, thanks to the precise cavity length control, the 5-µm aperture VCSEL achieved a WPE of 21.1 percent, marking a significant achievement.
“Just like a highly accurate scale enables the construction of detailed shelves, the precise use of in situ thickness control of GaN layers combined with thickness calibration of ITO electrodes and Nb2O5 spacer layers enables highly controlled fabrication of VCSELs, representing a powerful tool for obtaining high-performance and highly reproducible GaN-based VCSELs for highly efficient optoelectronic devices,” concludes Takeuchi.