MoS₂ offers new way to detect signals in cells
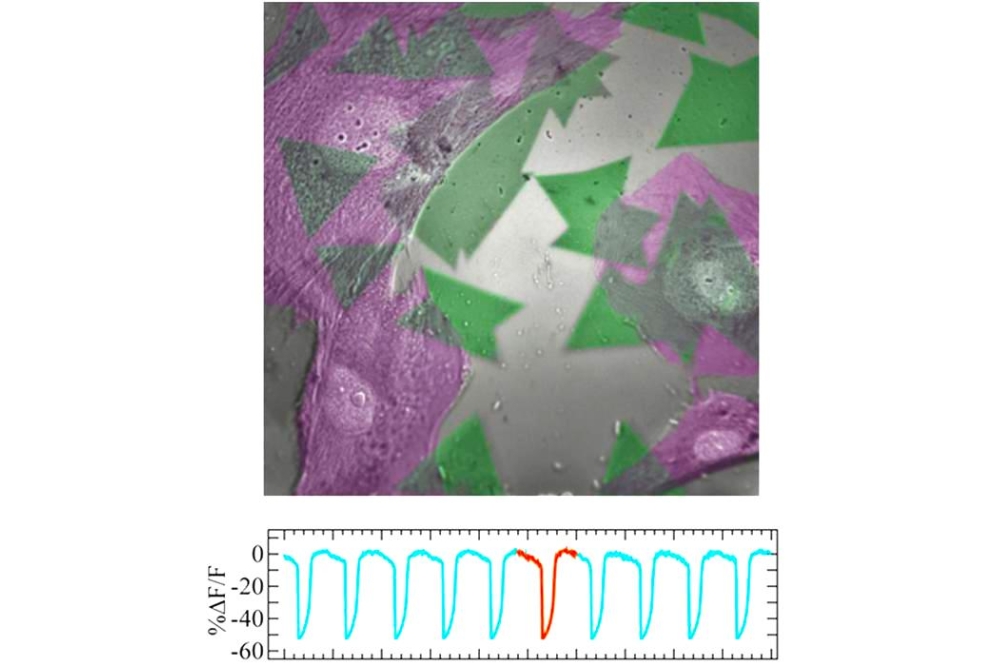
Scientists would like better ways to track the electrical activity of the body’s most excitable cells, such as neurons, heart muscle fibres and pancreatic cells. These tiny electrical pulses orchestrate everything from thought to movement to metabolism, but capturing them in real time and at large scales has remained a challenge.
For decades, scientists have relied on electrodes and dyes to track this electrical activity. Now, engineers at the University of California San Diego have discovered that the monolayer compound semiconductor MoS2 can do the job using light.
They show this in their study 'Trionic all-optical biological voltage sensing via quantum statistics' just published in Nature Photonics.
Traditional electrophysiology, which relies on invasive microelectrodes, offers precise recordings but is limited in scalability. Implanting electrodes across large regions of tissue can cause significant damage, and even the most advanced probes are limited to recording just a few hundred channels at once.
Optical techniques like calcium imaging, while capable of monitoring large populations of cells, offer only an indirect glimpse into electrical activity. Instead of recording the actual voltage shifts that drive cellular communication, they capture secondary changes that can introduce significant discrepancies.
The UC San Diego engineers have demonstrated a high-speed, all-optical method for recording voltage changes using ultra-thin semiconductors.
The key lies in how these materials’ electrons interact with light. When exposed to an electric field, they switch between two states—excitons (electron-hole pairs that are electrically neutral) and trions (charged excitons).
The researchers found that the conversion from excitons to trions can be harnessed to detect the electrical signals of heart muscle cells—without the need for tethered electrodes or voltage-sensitive dyes, which can interfere with cellular function.
“We believe that the voltage sensitivity of excitons in monolayer semiconductors has the potential to enable high spatiotemporal investigation of the brain’s circuitry,” said study senior author Ertugrul Cubukcu, a professor in the Aiiso Yufeng Li Family Department of Chemical and Nano Engineering, as well as the Department of Electrical and Computer Engineering, at the UC San Diego Jacobs School of Engineering.
Cubukcu and his team studied the quantum properties of monolayer MoS2. In addition to its biocompatibility, they found that this semiconductor material possesses a particular advantage: it naturally forms sulphur vacancies during its production, which creates a high density of trions. This built-in defect makes it exceptionally responsive to changes in nearby electric fields, including the ones generated by living cells, which in turn allow spontaneous exciton-to-trion conversion.
By tracking changes in the material’s photoluminescence, the researchers could map the electrical activity of heart muscle cells in real time, at speeds unmatched by any other imaging technology to date, the researchers noted.
This technology has a variety of potential applications. It could enable researchers to map network dysfunctions across large areas of excitable tissue, from the surface down to deeper layers. It could provide insights into the mechanisms underlying neurological and cardiac disorders, offering a clearer picture of how diseases disrupt the body’s electrical circuits.
It may also refine therapeutic strategies that rely on electrical neuromodulation, such as deep brain stimulation for Parkinson’s disease or cardiac pacing for arrhythmias. Furthermore, this work could lead to the discovery of new quantum materials that can offer a non-invasive, high-speed method to probe electrical activity in living systems.
Pictured above: Top: Coloured SEM image of heart muscle cells (green) cultured on top of monolayer semiconductor crystals (purple). Bottom: Optical recording traces of the cellular action potentials