
An abundance of key elements
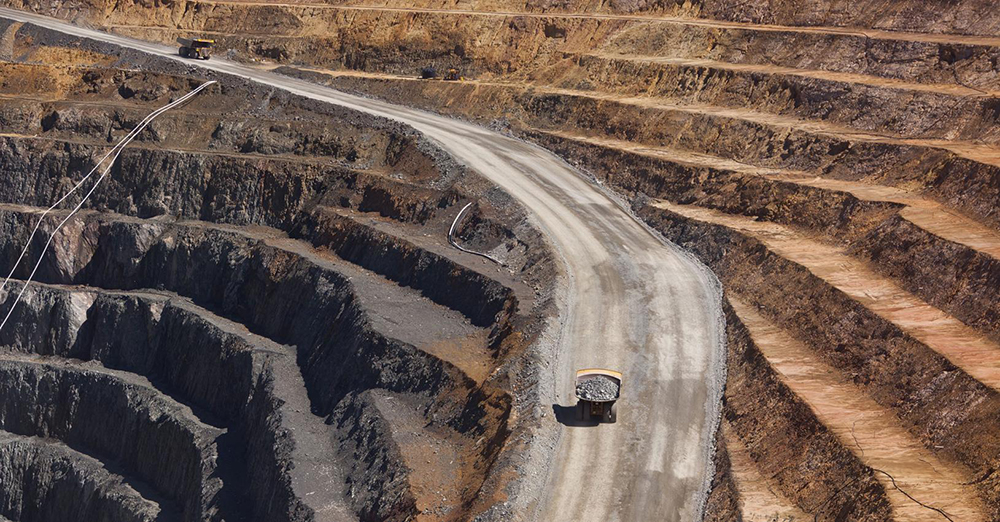
If demand for GaAs, InP and germanium substrates grows, it can be fulfilled, thanks to sufficient availability of gallium, germanium, and indium
BY MAX FRENZEL FROM THE HELMHOLTZ INSTITUTE FREIBERG FOR RESOURCE TECHNOLOGY
We tend to think of the substrate as the starting point for chip
production. Much thought is given to the selection of its size,
orientation, and doping level; there can be haggling over its price; and
it is the foundation for the growth of epilayers that form the heart of
the device.
But there is another way to look at this, where the substrate is not the starting point. Instead, the production of any chip begins further back, with the mining of raw materials, and the extraction of elements that are used to produce substrates and metal-organics.
Viewed in this light, a first glance would suggest that our industry is in a rather precarious position. That's because many of our key elements "“ including gallium, indium and germanium "“ are not the primary focus in mining operations. Instead, they are produced exclusively as by-products, extracted from the ground when mining major industrial metals. For example, the mining and processing of aluminium ores accounts for most gallium production. The by-products are worth far less than their host materials, so they do not impact mining or production decisions "“ and their primary production is strongly limited by the production of the host materials.
Nevertheless, over the past decades, the primary production of many by-products has been growing far faster than that of their corresponding hosts (see Figure 1). This raises the question of how sustainable such accelerated growth is. Any slowing would be a concern, as it could potentially apply the brakes to a ramp up in chip production.
The most common approach to evaluating the stocks of industrial metals is to determine the levels of reserves and resources. But when it comes to by-products, this approach is flawed. Instead, the amount of available by-product is determined by what can be recovered from the extracted host-ore streams. This is not well-known, but while the primary production of some by-products lagged the theoretical capacity in the 1970s, this is not necessarily true today.
A reliable figure for how much gallium, germanium and indium is available cannot be determined with back-of-the-envelope calculations. Instead, it requires a more sophisticated approach, considering both the supply potential and the supply curve, terms that will be explained in due course.
Taking this tack, our team at the Helmholtz Institute Freiberg for Resource Technology has found that plenty of affordable gallium, germanium and indium should be available for the compound semiconductor industry for the foreseeable future.
Figure 1. Relative growth in the primary production of gallium, germanium and indium from 1973 to 2013, compared to their corresponding host materials. Reproduced from M. Frenzel et al. Resour. Policy 52 327 (2017).
Supply potentials
To determine the availability of a by-product, one must know its supply potential. This corresponds to the amount of by-product that can be economically extracted per year from the ongoing primary production of relevant host ores, depending both on price and extraction technology. Due to these economic and technological constraints, the supply potential is always far less than the total amount of the by-product contained in host-ore streams.
Whether it is worth extracting a by-product from a given batch of host ore is governed mostly by its concentration. Essentially, extraction is only feasible above a certain threshold concentration, the so-called cut-off grade. The concentrations of different by-products vary significantly across different mines, so not all mines are suitable sources for a given by-product (for example, see Table 1, listing compositions of zinc concentrates from different mines). Furthermore, mines also vary greatly in terms of their annual production volumes.
Due to the innate heterogeneity of geological materials, and variations in the size of mines, it is impossible to produce a sensible estimate of the supply potential by multiplying the total annual volume of a host-ore stream with an average concentration of the by-product, and perhaps an estimated recovery. Instead, supply potentials must be calculated on a mine-by-mine basis. The sum over all relevant mines then gives the global total.
Unfortunately, it is not quite as easy as that in reality. The stumbling block is the general lack of publicly accessible data on the composition of mined ore concentrates. Due to this, supply potentials can only be assessed probabilistically. We have approached the task in this manner. Using probability distribution functions for concentrations of by-products in host ores from different geological types of mines, we have constrained the probable range of supply potentials with Monte Carlo simulations.
Table 1. Gallium, germanium and indium in zinc ore concentrates from different mines. Data from M. Frenzel et al. Ore Geol. Rev. 76 52 (2016).
Estimates of the probability distribution functions were made using analytical data from the scientific literature. We considered the following host ores: bauxite (gallium), sulphidic zinc ores (gallium, germanium, indium), coal (gallium, germanium) and sulphidic copper ores (indium). This approach has much merit, as it closely mirrors current primary production sources.
Estimated supply potentials for gallium, germanium and indium are shown in Figure 2, showing that they significantly exceed current production. According to our results, gallium and germanium production could increase at least five-fold and indium production could double, assuming factors such as price and technology remain the same.
This begs the question of why there is such a large discrepancy between current production and supply potentials. Primarily, it results from a lack of extraction capacity at the smelters. Here, the focus is on the core business of extracting the main products, so investment tends to be directed at either increases in main-product capacity or efficiency improvement, rather than improving the extraction of by-products.
Another factor at play is the limited demand for these by-products. Their markets are relatively small, so the installation of extraction capacity at a large smelter could have unwanted consequences: it could easily result in oversupply, driving down prices and leading to financial losses for the smelter.
Such a scenario is by no means inconceivable, given the sensitivity of these markets. This is illustrated by the collapse of Fanya Metal Exchange in China in 2015 that led to a tumbling of the indium price. The collapse eliminated a relatively large buyer, creating a considerable oversupply of indium, and ultimately depressing prices in recent years. If a new producer entered the market, a similar effect could take place.
Figure 2. Probability distribution functions, based on Monte Carlo simulations, describing estimated supply potentials of gallium, germanium and indium relative to current primary production (for the year 2014). The probability distribution functions are normalised to the current primary production of these elements. Note that a value of 2 on the x-axis corresponds to about double current primary production. (Graphs adapted from M. Frenzel et al. Resour. Policy 52 327 (2017)).
Future availability
Supply potentials vary with market conditions because they depend directly on the interplay of concentration, extraction technology and market price. Therefore, stationary estimates of supply potentials are of limited use for considerations of future availability.
Producing supply curves for gallium, germanium and indium allows us to understand how availability might evolve in the future. These plots, examples of which are given in Figure 3, show how higher market prices can support extraction from lower-grade materials, and therefore correspond to higher values of the supply potential. Any improvements in technology will shift the curves downwards, since they lower extraction costs. This also equates to an increase in supply potential, but at constant prices.
The supply curves have an inelastic region where the price does not affect the supply potential (the vertical parts of the curves). When considering future availability, it is important to know how far the current supply potentials are away from this inelastic region. According to Figure 3, gallium from bauxite is clearly in the elastic region, while germanium and indium (from sulphidic zinc ores) are very close to the inelastic part. The implication is that it should be easy to increase the gallium supply potential in the future, and more difficult to increase supply potentials for germanium and indium. As indium also has a more limited current supply potential than the other two, it will be the hardest to provide a major increase in future production.
This situation could change markedly if there is a significant hike in the price, making the by-product as valuable as the main product in the corresponding host ores. In this scenario, denoted by the "˜break-down points' in Figure 3, the by-product supply curves cease to apply, with higher production coming from mines producing these elements as their main products. It is worth noting that the break-down points only occur well into the inelastic supply regime. The implication is that it would take a significant price-hump to expand production beyond by-product limits.
Implications
A key finding emerging from our work is that there are currently no issues of availability associated with gallium, germanium or indium. What's more, given the relatively large potential for future increase in the supply of these elements, it is improbable that such issues will develop over the next decade. If there are to be any constraints in the longer term, they will most likely arise with indium, due to its comparatively limited capacity for increases in primary production.
Our findings may be integrated into product design studies that assess whether raw material availability has the potential to constrain the market penetration of a particular product. Consider, for example, one of the biggest potential consumers of indium: the copper-indium-gallium-selenide (CIGS) thin-film solar cell. This class of photovoltaic, which has a current maximum conversion efficiency of about 20 percent, typically requires 2.5 g of indium for every square metre. Strengths of these cells include a lower cost than traditional crystalline silicon and less energy intensive production. Of the four elements in the device, indium has the lowest supply potential.
To prevent major climate change, about 4000 GWp of photovoltaic capacity must be installed by 2040, according to the International Energy Agency (IEA). This equates to 20,000 km2 of cells with an efficiency of 20 percent. So, if CIGS cells were used exclusively to hit this target, 175 GWp of them would have to be installed each year. This would require 2,200 tonnes of indium per annum.
Is this feasible? Well, 2,200 tonnes is about three times current primary production, and about 50 percent higher than the current minimum supply potential of indium, which is 1,500 tonnes per year (cf. Table 2). Note also that there are other applications that consume a significant amount of indium.
Figure 3. By-product supply curves of gallium, germanium and indium from different raw materials for the year 2014. The extractable amount is ultimately limited by the total amount of by-product contained in host ore streams. This is reflected in the sharp rise towards the right-hand side of the curves. The vertical part of the curves is called inelastic. Data is taken from M. Frenzel et al. Resour. Policy 47 38 (2016); M. Frenzel et al. Resour. Policy 46 45 (2015); and M. Frenzel et al. Resour. Policy 52 327 (2017).
Consequently, one must conclude that CIGS technology may not be capable of providing the lion's share of the required solar panel installations. However, it can still have a role to play: the availability of indium is high enough to support a significant share (tens of percent) of this technology in the global photovoltaic market.
While gallium, germanium and indium may be the most important metals in the compound semiconductor industry, they are not the only ones. For example, there is also tellurium, the group VI metal used in long-wavelength detectors. It might have a significantly more limited supply potential, but this is hard to tell, because there is currently a lack of reliable quantitative data.
Consequently, there is more work to do.
Table 2. The global supply potentials of gallium, germanium and indium in 2014. Data from M. Frenzel et al. Resour. Policy 52 327 (2017).