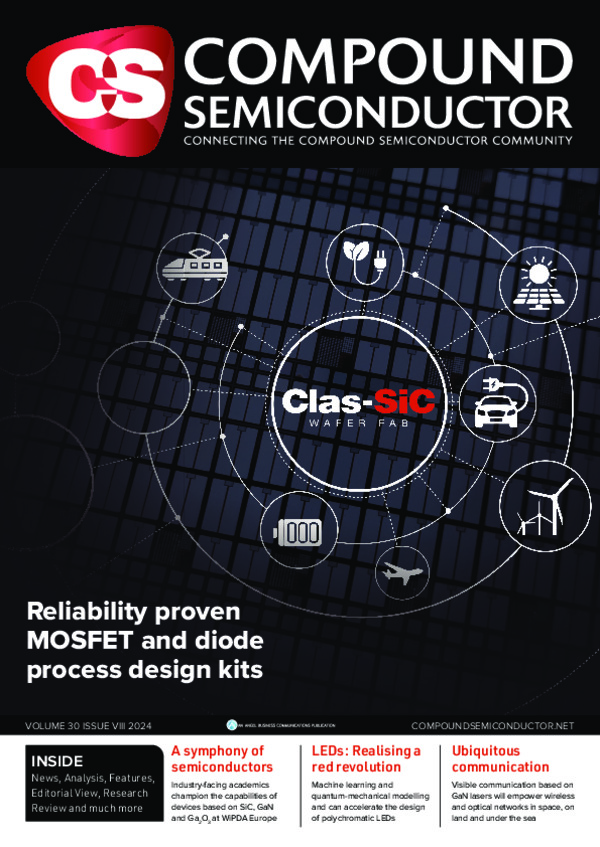
Targeting ubiquitous communication with GaN lasers
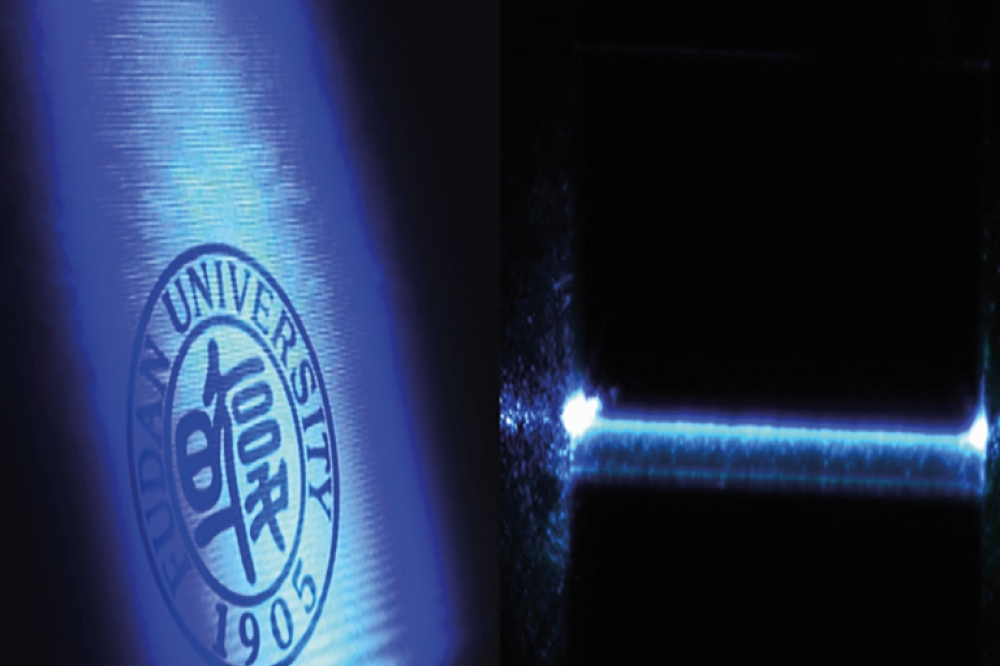
Visible communication based on GaN lasers will empower wireless and optical networks.
BY CHAOWEN GUAN, NAN CHI AND CHAO SHEN FROM FUDAN UNIVERSITY
While science fiction often falls short of depicting the current capabilities of technology, it still offers a glimpse into potential future advancements. A prime example is Marvel Comics’ hero Iron Man, whose body armour has evolved since its debut in 1962. In recent iterations, Tony Stark’s suit features nanoscale devices that communicate with one another. For science fiction to become science fact, such body armour would require formidable capabilities in high-speed data transmission.
Although we are unlikely to try and exactly mimic Iron Man, there are moves to more wearables, along with the rapid development of technology for 6G wireless data links. Within this infrastructure, visible light communication (VLC) is poised to play a crucial role. VLC is expected to provide wide-coverage communication across space-air-ground-underwater domains at ultra-high speeds and low latency. In the future, light could even be used to power the Internet of X-things.
What is VLC?
The term ‘visible light communication’ is relatively self-explanatory. It refers to a wireless optical transmission technology that utilises visible light for data communication within the spectral range 380 nm to 790 nm.
In stark contrast to traditional wireless communication, which grapples with a scarcity of spectral resources, visible light communication enjoys an abundance, boasting a bandwidth of around 400 THz. This vast expanse of untapped potential beckons human exploration and innovation. Additional strengths provided by VLC are an immunity to radio interference, an absence of electromagnetic radiation, and high confidentiality. However, the biggest advantage of VLC is its high speed. Today’s lab record for data transmission stands at over 20 Gbit/s, a rate so high a wireless download of a movie in the Ultra HD format takes just a few seconds.
A typical VLC system comprises three main parts: a visible light transmitter, a channel for transmission, and a receiver. Information transfer begins with encoding, modulating, and pre-balancing the signal in the optical transmitter. Transmission then occurs through free space to reach a receiver, where either a p-i-n photodiode or an avalanche photodiode converts the optical signal into an electrical one. This is followed by equalisation, demodulation, and decoding to restore the original transmission signal.
Today there are two significant limitations with VLC systems. One is associated with the transmitter, such as a high-speed blue laser, in intensity-modulation direct-detection systems. This soure is held back by a relatively small bandwidth, as well as a level of maturity that falls far short of its counterparts operating at around 1550 nm. The second limitation is the slow development of key components, such as external modulators, amplifiers and balanced photodetectors in coherent modulation systems.
At Fudan University we are striving to address these all limitations, initially by developing a novel GaN laser that offers a higher modulation rate.
(a) Laser epitaxial structure. (b) Microscopic photographs of the high-speed blue laser diode chip.
High-speed sources
As the ancient Chinese saying goes: A craftsman who wishes to do his work well must first sharpen his tools. We have taken this advice to heart in developing a GaN-based laser, a fundamental component for white light and a promising source for underwater wireless optical communication. At present, GaN-based lasers emitting in the purple, blue, and green are primarily deployed for the likes of optical storage, lighting, displays, and material processing, with a focus on DC characteristics, such as output power and slope efficiency. Since there is a strong built-in electric field presented in blue lasers fabricated on c-plane GaN substrates, owing to polarisation effects, researchers are utilising semi-polar and non-polar GaN substrates to eliminating such polarisation fields. This enables to a better efficiency and a higher modulation bandwidth. However, the supply of GaN substrates with suitable orientations is limited.
Our efforts at developing high-speed GaN-based lasers have considered both the design of the epitaxial structure, including the active region and the electron-blocking layer, and the device architecture, such as the ridge waveguide. Through simulation of various epi-structures we have uncovered design rules that have led us to an improved frequency response in InGaN/GaN quantum well lasers, with findings subsequently verified by experimental work.
Measurement and characterisation of our high-speed lasers has involved the detailed analysis of light-current-voltage characteristics, emission spectra, junction temperature, relative intensity noise, frequency response, and data transmission performance. We have obtained the threshold current and slope efficiency of our lasers by determining light-current-voltage characteristics. The intrinsic parameters of our lasers have been further extracted. The -3dB modulation bandwidth can be derived through small-signal frequency response testing. During this work we gained much insight into the influence of design on performance – for example, the influence of different waveguide layer structures, with thicker ones found to lead to a more divergent light field that increases its overall volume. The optimal thicknesses for the p- and n- waveguide layers are 50 nm and 150 nm, respectively.
VLC application scenario.
As is the case for the LED, shrinking the dimensions of the laser diode offers a promising route to improving its high-speed operation. We have found that a shorter cavity enhances the modulation performance, thanks to a smaller optical field volume. However, this comes at a cost, with increased mirror loss and a suppression of the damping effect. Despite these drawbacks, we have demonstrated a high-speed blue laser-based VLC system achieving a transmission rate as high as 20.06 Gbit/s, and a variant with green lasers reaching 17.73 Gbit/s.
Building on the rapid development of laser-based VLC technology, we are refining this technology for a world with better connectivity. Our vision for VLC includes indoor systems that combine lighting and communication, as well as long-distance and underwater wireless optical communication. To turn these ideas into reality, our lab is pursuing innovative designs and practices.
VLC for mobile…
When laser diodes are deployed in VLC systems, they promise a high modulation bandwidth and output power, attributes that can lead to high-speed, long-distance communication. However, as laser diodes produce a narrow beam, pointing and alignment are required in laser-based optical wireless links, which limits the mobility of communication systems. VLC with a wide coverage transmitter and enhanced mobility offers a fundamental advantage for wireless services by providing users with flexibility and convenience, while enabling connections while on the move. Therefore, we go beyond a point-to-point VLC link and are trying to figure out a way to address mobile-communication-related challenges.
Progress has come from leveraging a laser-based light transmitter with wide spatial coverage, enabling a VLC system with a mobile receiving end. Using on-off keying (OOK), which is the simplest modulation technology, a data rate beyond 500 Mbit/s has been achieved in laser-based white light VLC link. Transmission is stable at distances of both 1 m and 2 m, and the system is capable of supporting a transmission rate of 500 Mbit/s when it’s moving at 0.48 m/s. Although distance and mobility impact the system’s performance, it is able to maintain a considerable degree of reliability and efficiency.
The design of a wavelength-division multiplexing VLC system, and the corresponding physical prototypes.
… indoor illumination…
One key advantage of VLC is that it can combine illumination and communication. To ensure that this is realised with a low carbon footprint and reduced operating costs due to compatibility with lighting systems, VLC may not come from increasing the number of light sources. Instead, it is a good idea to expand the field-of-view of a single light source.
A white light source is designed by combing a blue laser array, a diffuser, a plano-convex lens, and a ceramic phosphor plate. The laser-based white-light source exhibits excellent stability, with an irradiation range extending to a half-angle of 20 °. To ensure high spectrum-utilisation efficiency, we employ discrete multi-tone bit-loading modulation. With this approach, we divide the laser’s bandwidth into 256 sub-carriers, each matching the modulation order based on its bit error rate. Using this transmitter in a VLC system, a peak data transmission rate of 3.24 Gbit/s has been achieved, spanning 1 m to 5 m. The results are promising for demonstrating a long-distance, wide coverage, high-speed VLC link for mobile network applications, as well as the feasibility of utilising a white-light emitter based on a laser for solid-state lighting.
… and satellite interconnects
Up in space, one important trend is the building of satellite constellation. This has implications for real-time data links between multiple satellites that require communication methods with high capacity, low latency, and high reliability. Laser communication is often used between satellites. Currently, infrared band is chosen for inter-satellite and satellite-to-ground communication, due to the matured devices and systems. Moving forward, given the radiation hardness of GaN and its associated alloys, the blue laser might be a useful source for inter-satellite optical wireless communications, such as those involving low-earth orbit satellites to provide internet access.
To advance inter-satellite communication via VLC, we have focused on increasing communication capacity and distance. For the former objective, we have been investigating the capability by using wavelength-division multiplexing (WDM) technology. Using a 40-channel WDM VLC system, we have achieved a total transmission rate of 418.3 Gbit/s, employing discrete multi-tone bit power loading and a Levin Campello algorithm. In terms of increasing the communication distance, we applied bidirectional reservoir computing to a VLC waveform for symbol-level equalisation, an approach that enabled us to record transmission at 11.2 Gbit/s over a free-space distance of 100 m.
Video streaming underwater
Another important application of VLC is underwater wireless optical communication (UWOC). Due to the presence of a blue-green transmission window in seawater, lasers emitting in this spectral domain are attractive candidates for enabling an underwater wireless data link.
At present, the main challenge facing UWOC is the prevalent time-varying and complex channel characteristics. A typical laser-based UWOC link is based on Gaussian beams. When disturbances such as bubbles, turbulence or obstacles are present, the quality of the beam reaching the receiver end degrades. Typical issues include a decrease in light intensity and a violent drift motion of the beam centroid. As a result, delivering stable, high-speed data transmission over long distances is challenging.
Our team is addressing this challenge by introducing a high-efficiency visible ultra-broadband auto-focusing Airy beam meta-surface. In the self-focusing area, the obstruction caused by small-scale obstacles is reduced by the energy carried in the sidelobes; and in the divergent region, the unique circular energy distribution of the Airy beam enhances the system’s adaptability to obstacles. Thanks to this meta-surface design, which exhibits a high polarisation conversion efficiency over a broad spectral range spanning 440 nm to 640 nm, the data transmission rate of wireless optical communication systems is increased by 91 percent, enabling reliable 4K video transmission via WDM using underwater optical communication data links.
Looking ahead, there are still many challenges to overcome in laser-based VLC. Much progress is required to propel the modulation bandwidth of GaN-based laser diodes to tens of gigahertz. While the telecommunication community is focusing on optical links operating at 100 G bit/s to Tbit/s, researchers in the VLC community are just entering the game, with data rates of tens of Gbit/s.
Airi Light under water optical communication system based on a
meta-surface. (b) Underwater 4 K video streaming using the full-colour
meta-surface system. (c) Received video frames with and without the
full-colour meta-surface at different frame sequences.
Moreover, today’s VLC systems are employing intensity-modulation direct-detection. This is because external modulators, amplifiers, and other important optical communication components in the visible spectrum are still missing. Meanwhile, the development of coherent VLC systems has just started, calling for the introduction of narrow linewidth lasers and high-speed balanced photodetectors.
In our efforts to advance VLC, our team is working with global partners to develop high-performance III-nitride optoelectronic devices and visible light photonic integrated circuit technology. Together with international collaborators, we are striving to realise an environmentally friendly, energy-saving, low-cost, and high-speed laser-based VLC technology as a vital component of the 6G network.