
Weighing up the options for gallium oxide crystal growth
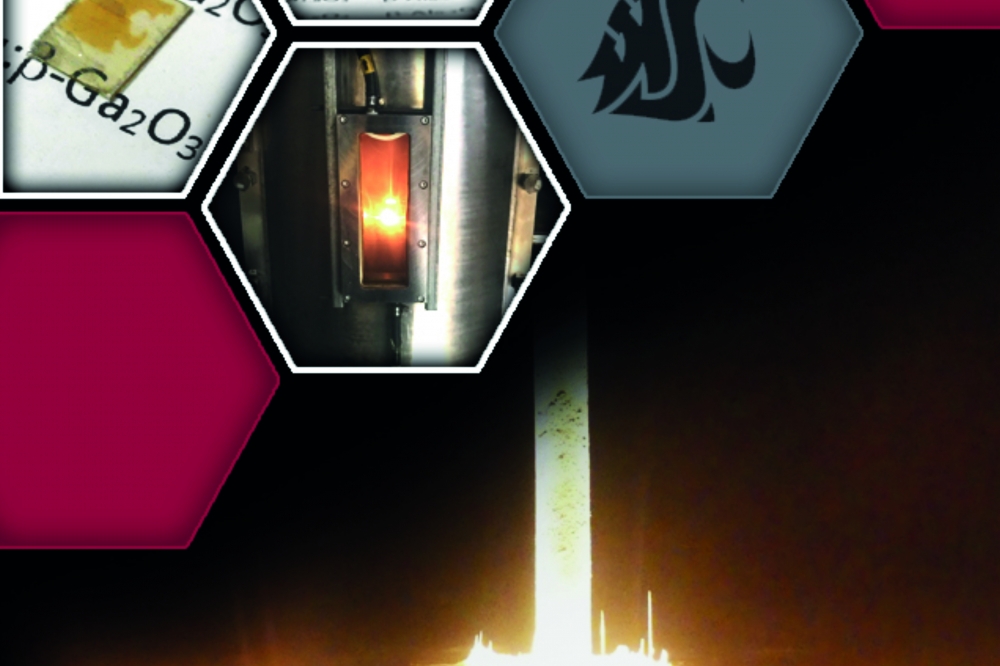
What are the pros and cons of producing β-Ga2O3 by the two most common techniques, the Czochralski method and edge-defined film-fed growth?
BY JANI JESENOVEC AND JOHN MCCLOY FROM WASHINGTON STATE UNIVERSITY.
There’s much excitement surrounding ultra-wide bandgap materials, such as Ga2O3, AlN and diamond. They are renowned for a very high intrinsic voltage breakdown field, which makes them exceptional candidates for handling high powers.
Within this family of semiconductors, interest in Ga2O3 is on an exponential trajectory. As well as its great potential for making incredibly small and efficient power-switching devices that trim thermal losses, it is attracting attention for the fabrication of deep-UV detectors that are transparent to visible wavelengths.
For developers of all forms of Ga2O3 devices, a key decision is the choice of polytype. There are many to decide among, but arguably the most important of all is β-Ga2O3, the room-temperature stable phase. One of its most promising features is that it can be alloyed with Al2O3 to increase the bandgap and push the transmission window deeper into the UV.
Efforts at understanding the nature of β-Ga2O3 rely on the growth of high-quality material. This can be in the form of a bulk single crystal, or a thin film that is grown by one of many methods. Today, high quality single crystal films of β-Ga2O3 may be deposited by MOCVD, HVPE and MBE.
One of the merits of bulk β-Ga2O3 is that, unlike the other ultra-wide bandgap materials, it can be formed from the melt, using techniques such as the Czochralski crystal growth technique, which has been employed for the manufacture of silicon wafers for many years. Crystals of this oxide can also be produced by edge-defined film-fed growth, a technique employed for commercial production of β-Ga2O3. In addition, research is underway into the growth of β-Ga2O3 by other methods, such as the vertical Bridgman and optical float zone techniques.
In the remainder of this feature we shall review the progress made with the more common methodologies and detail insights provided by characterisation. Within this survey, we include a brief account of the contribution to this field by our team from the Institute of Materials Research at Washington State University.
Of the techniques we have mentioned for crystal growth, the two primary approaches – the Czochralski method and edge-defined film-fed growth – have much in common. They both involve pulling solid single crystals out of molten Ga2O3, held at temperatures above 1800 °C and housed in iridium metal crucibles. Ga2O3 produced by the Czochralski method tends to be cylindrical, while that formed by edge-defined film-fed growth involves the use of iridium capillaries, which can draw the material into the desired shape.
Pioneering β-Ga2O3 produced by the Czochralski method is a team at the Leibniz Institute for Crystal Growth, Germany. Commonly known as IKZ, this institute has demonstrated marked success, detailed in many publications and several patents.
Famous for producing β-Ga2O3 via edge-defined film-fed growth is Novel Crystal Technologies (NCT). This Japanese outfit, which manufactures and sells wafers of doped or un-doped β-Ga2O3, touts that crystals produced by this technique are superior to those made by the Czochralski method, due to the potential to produce very large wafers. However, edge-defined film-fed growth usually forms thin crystals, so the overall pulled volume is lower than that associated with the Czochralski method, which has the potential to scale and yield large cylindrical single crystals.
Delving into doping
For all semiconductors purity is critical. When small concentrations of other atoms are present, or defects added, this strongly affects the bulk properties. In the case of β-Ga2O3, there is the opportunity to intentionally replace the gallium that sits on its atomic site with other elements, via doping, to induce optical or electrical phenomena; and there is the threat that undoped β-Ga2O3 can be plagued with impurities. Common impurities in β-Ga2O3 produced by any method include silicon, iron, and chromium; iridium may also be introduced when crystals of this oxide are formed by the Czochralski method or edge-defined film-fed growth. Metallic impurities tend to originate from the precursor powders used in synthesis, or from the crucible that can contaminate the melt at high temperatures. Due to this, undoped β-Ga2O3 is referred to as ‘unintentionally doped’ material. Typically, this contains substantial background impurities, acting as dopants and enabling electrical conduction.
Intentional doping of β-Ga2O3, a topic undergoing much research, offers control over the electronic behaviour of this oxide. Material that is n-type, and thus dominated by electron conduction, may be formed by doping with silicon, germanium, tin, zirconium or hafnium. If electrically insulating behaviour is desired, appropriate dopants include iron, magnesium, zinc, nickel and copper. Realising p-type behaviour has proved far trickier. Some annealing experiments suggest marginal p-type behaviour may be realised due to hydrogen interaction, but this behaviour has not been observed in as-grown bulk crystals. Maybe this is not surprising, given that this difficulty has been observed in ZnO, another transparent semiconducting oxide. In the case of ZnO, this weakness has eventually limited the application of the material.
A property that may pique the interest of many is photodarkening, which has been demonstrated in copper-doped β-Ga2O3 by the Institute of Materials Research at Washington State University. Uncommon in semiconductors, this novel effect is long-lived in copper-doped β-Ga2O3, with UV excitation causing a sample held at room-temperature to darken and remain in that state for weeks (see Figure 2). Note that heating accelerates the reversal of this darkening.
Figure 1. Exterior view of insulation and the growth chamber as gallium oxide is pulled from the melt with the seed rod (centre), while temperature is monitored with a movable pyrometer (left).
Growing pains
The progress that is been made with β-Ga2O3 crystal growth allows researchers to access substrates, deposit thin films on them, and investigate devices. But it does not follow that no further progress is required in crystal growth. Today, growth of β-Ga2O3 is very expensive, partly because iridium prices are very high, and the crucibles made from this metal weigh upwards of 0.5 kg.
Progress may come through a switch to growth in other crucibles. With the vertical Bridgman technique it is possible to use a platinum-rhodium alloy. A popular choice for growing other semiconducting materials is molybdenum – but this is unlikely to yield much success, due to the rapid decomposition of β-Ga2O3, which oxidizes and destroys the crucible.
The team at IKZ continues to make advances with the Czochralski method, including the scaling of material to diameters of up to 5 cm and lengths of 6 – 8 cm. One of the issues that they face, along with anyone else that is trying to use this growth method, is a lack of stability that stems from the dopants. The addition of elements such as silicon, zirconium, hafnium and tin – added to induce conductivity – leads to absorption of radiation in the infrared, associated with excess electrons. Allied to the relatively low thermal conductivity of β-Ga2O3, this absorption is behind the retention of large amounts of heat during growth. That’s far from ideal, as crystal growers rely on a highly tailored temperature gradient between the melting point of the material in the crucible in liquid form, and the temperature of the pulled crystal. Due to the near-IR absorption associated with free-carrier absorption, the melt-crystal interface can become unstable and form a corkscrew shape, rather than the typical cylinder. Corkscrews arise when the material dynamically forms geometries capable of dissipating heat. To prevent this from happening, the length of doped β-Ga2O3 crystals produced by the Czochralski method must be limited compared with their insulating or un-doped single-crystal siblings that do not have free-carrier absorption.
Several strategies have been employed to mitigate spiral growth of conductive β-Ga2O3. By turning to alloying with aluminium, we have improved crystal stability (see Figure 3).
Figure 2. A Washington State University cougar head logo
photo-darkened through a mask onto a copper-doped sample with a 275 nm
LED, where it remains for several weeks before returning gradually to
the original homogenous colour.
Substrates obtained from crystals produced by the Czochralski method are typically (100) oriented. However, it’s also possible to produce polished (010) and (001) samples. These crystallographic orientations are highly valued, due to the asymmetric repeating-lattice monoclinic structure of β-Ga2O3 that is behind electronic and optical properties, which are governed by the particular crystal orientation.
It is easier to grow conductive β-Ga2O3 by the edge-defined film-fed method. That’s because the iridium capillaries force the single crystal to retain its shape when it is pulled. NCT applies this technique to its commercial production of tin-doped β-Ga2O3. The material that is produced by this method tends to have similar orientations to that of Czochralski crystals. However, the (201) orientation is also available via edge-defined film-fed growth – this is much more difficult to obtain via the Czochralski method, due to the nature of this growth technique. The (201) orientation has some technical challenges, with crystallographic twins forming in edge-defined film-fed growth. But these twins are not commonly seen in material made by the Czochralski method.
Options for characterisation
Within the scientific community, much effort has been devoted to characterising β-Ga2O3, with researchers undertaking electrical, optical, structural, and even magnetic measurements of this oxide. High-resolution rocking curve X-ray diffraction is widely used to analyse the structure of single-crystal β-Ga2O3, with the results supplied on commercial wafer specifications as a certificate of quality. These measurements often consider the (400) or (600) reflections of the crystal lattice, to determine whether there is any asymmetry in the material or knees to the curve. If these features appear in the curves, this reveals that the material is impaired with grain boundaries or twins, as well as a poor surface quality. For single-crystalline wafers and substrates, the full-width at half-maximum of the diffraction peaks ranges from 20 arcsec to 150 arcsec.
Other approaches are needed to uncover point defects, inclusions and inhomogeneity. A common tool for this is scanning or transmission electron microscopy, which may be used to study the atomic structure of samples, locate point defects and analyse inclusions and homogeneity.
Like many other semiconducting materials, the common approach for studying the surface roughness of bulk single crystals, prior to growing thin films upon them, is atomic force microscopy. This technique is incredibly insightful for assessing the impact on surface roughness resulting from polishing, chemical etching and annealing.
It is crucial to be able to determine material impurities in β-Ga2O3, because as well as leading to insulating or n-type conducting behaviour, these additions can lead to luminescence – that’s the case for chromium and magnesium. Fortunately, many techniques can uncover impurities, including mass spectrometry and a number of optical emission spectroscopy techniques such as: glow discharge mass spectrometry; inductively coupled plasma mass spectrometry; secondary-ion mass spectrometry; and laser ablation, inductively coupled plasma mass spectrometry.
The latter of these has proven to be highly useful for studies of the spatial differences in β-Ga2O3 doping and inclusions. This optical technique involves focusing a laser on a sample’s surface, using a beam width of typically around 10 µm. Due to the high intensity of the incident radiation, the laser beam ablates the surface or drills into it. The ablated material forms an extremely hot plasma, before ions are separated electromagnetically and counted, one by one, by a detector.
This laser-based technique has advantages over the common alternative, secondary-ion mass spectrometry, which requires expensive calibrated standards in the matrix being measured. The use of a laser enables rapid horizontal, vertical, and patterned measurements of substrates, can sample and identify inclusions, and is capable of detecting doping gradients. Another of its merits is that it can provide quantitative data, using simple geological standards to convert count rate into atoms per cm3. And even if standards are not available, this technique offers a qualitative assessment of counts, which is still useful to determine the location of an inclusion and its composition.
Two complementary techniques for studying β-Ga2O3 are Raman microscopy and photoluminescence. Both offer insight into behaviour that’s associated with doping and alloying, and facilitate forensic analysis of inhomogeneous materials. Additional information unveiled with Raman microscopy includes local vibrational analysis of inclusion phases, identification of lattice changes due to alloying, and help in ascertaining crystal orientation.
Light emission via photoluminescence can be obtained with some laser excitation Raman systems, or alternatively with a dedicated system. Detecting luminescence has much value, as it can be used to identify some impurities and dopants. Chromium, the active element in ruby laser crystals, acts as a very bright emitter in insulating β-Ga2O3 crystals; and the additional presence of magnesium can shift the chromium luminescence from red to orange.
Organic contaminants and certain other impurities, such as rare-earth elements, can also be identified by these techniques, which makes them excellent for comparing the surface of β-Ga2O3 crystals grown by a variety of approaches. For example, luminescence of chromium may be less intense in samples produced by the optical float zone process, because they don’t use crucibles, which typically leach this element. If substrates produced by either the Czochralski method or edge-defined film-fed growth are to be used for any application, they must first be carefully analysed for inclusions.
When studying transparent materials, such as Ga2O3, optical characterization tends to employ transmission spectroscopy that utilizes light from the UV to the IR. This approach allows researchers to identify absorption from dopants and impurities, and to verify the presence of free-carrier absorption, indicative of n-type material.
For determining the electrical properties of β-Ga2O3 that is n-type or unintentionally doped, the use of the Hall effect is ideal, providing values of electron volumetric concentration and mobility. Armed with this insight, one can assess the material’s electrical conductivity. For insulating materials, a lack of carriers hampers the use of the Hall effect, so it may be better to use other methods to assess resistivity, such as impedance or current-voltage measurements.
To study impurities, defects, and their energetic location within the ultrawide bandgap, researchers can turn to electrical defect spectroscopy techniques, such as deep-level transient spectroscopy and thermoelectric effect spectroscopy. Complementing these are techniques such as thermoluminescence spectroscopy, allowing optical analysis of defects.
Meanwhile, when there’s a need to determine how the concentration of defects varies with depth from the surface, one can turn to positron annihilation spectroscopy. This form of spectroscopy involves injecting positrons into the surface – they annihilate with electrons, with characteristics that depend on the open volume. Crucial information is revealed, especially associated with gallium vacancy formation in thin films or single-crystalline bulk samples.
Figure 3. A gallium oxide Czochralski boule, alloyed with alumina to increase the bandgap.
Looking ahead
Over the last decade, much progress has been made in the growth of unintentionally doped and insulating β-Ga2O3 bulk substrates. Efforts at IKZ have led to significantly larger, higher-quality crystals using the Czochralski method, and progress has been realised by NCT using edge-defined film-fed growth. These technologies are now mature enough to provide production-quality substrates, which can provide a foundation for thin-film growth or serve in other applications.
However, all this success should not lead to complacency. Much more needs to be done, including advancing the growth of conductive β-Ga2O3 by the Czochralski method and addressing issues associated with the length of crystals produced by this technique. Efforts in academic laboratories are being directed at research into novel dopant and alloy behaviour, with many institutions and individuals pushing the material into new applications and discovering interesting phenomena.
One critical issue is p-type doping. The concern is that β-Ga2O3 will mirror ZnO, which lacked a bulk dopant to induce p-type behaviour. If that’s the case, β-Ga2O3 may also be fundamentally incapable of high-mobility hole conduction. However, there’s good reason for cautious optimism, given that some experiments have shown marginal success, with annealing inducing p-type behaviour. Given the great promise of β-Ga2O3, let’s hope further success follows.
Main Image Credit: Jeffrey Jensen