
GaN diodes with uniform, robust avalanche
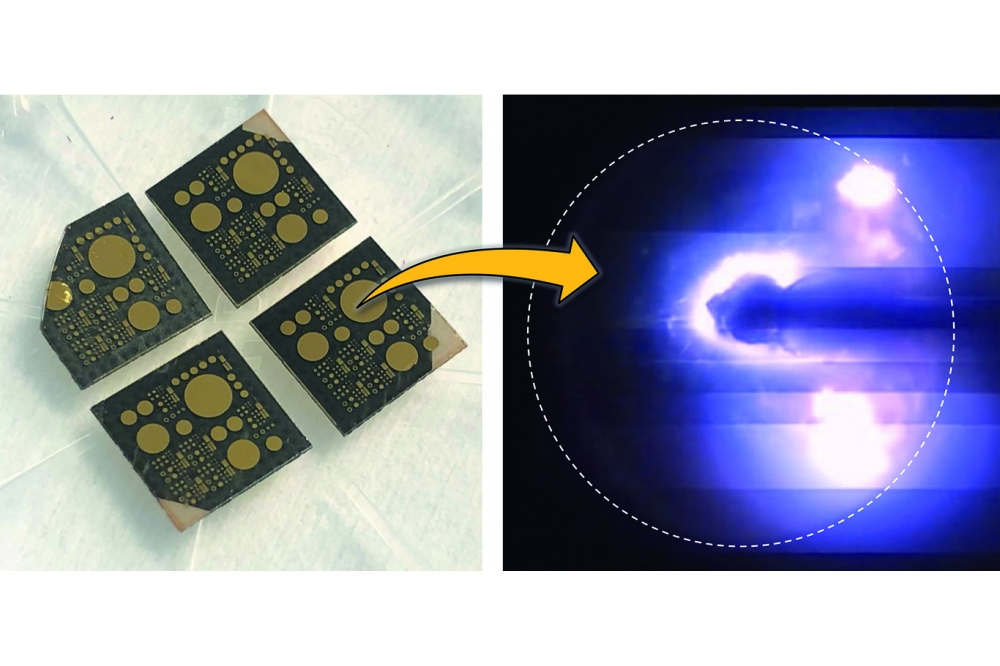
A multi-faceted endeavour, starting with material growth and extending
all the way to circuit-level investigation, ensures uniform, robust
avalanche in GaN vertical power diodes.
BY BHAWANI SHANKAR AND SRABANTI CHOWDHURY FROM STANFORD UNIVERSITY
In every power electronic system, it is the power devices that provide the building blocks. When these semiconducting devices, often diodes and transistors, are used in power electronic circuits, they are often placed in series with inductive loads, such as a motor or a discrete inductor.
In this configuration, when device switching occurs, there’s an interruption to current flow through the series inductor – and this generates a voltage transient, appearing across the device terminals (see Figure 1). If the voltage transient exceeds the breakdown voltage of the device, this poses a threat to damage the device.
To prevent failures from such overvoltage scenarios, device engineers tend to design power devices with extra headroom in the breakdown voltage. But there are penalties to pay: a higher on-resistance, leading to an increase in conduction losses; and a higher device manufacturing cost, stemming from a hike in semiconductor estate associated with the larger drift region volume needed to hold the higher voltage.
Combatting this concern are semiconductor devices with an avalanche capability, which have an inherent overvoltage protection. When such devices face overvoltage transients in a circuit, they undergo avalanche breakdown and generate micro-plasma or current filaments that bypasses the electrical stress and protect the device.
Fortunately, one of the most promising materials for the future of power electronics, GaN, possess avalanche capability. However, despite more than three decades of exploration of this wide bandgap semiconductor as a mainstream device material, reports of its avalanche breakdown are limited to devices that have been grown on native GaN substrates.
Figure 1. A power MOSFET in series with an inductor experiences voltage
overshoot during switching. If the FET is not avalanche capable, the
overvoltage can cause device failure. To avoid premature failures,
typically the device is overdesigned for a breakdown voltage (VBR) higher than required.
Achieving uniform avalanche
This limitation may raise a few eyebrows, given that any GaN-based device with an intrinsic p-n junction should show avalanche behaviour, so long as it has been designed correctly. But there are valid reasons why there are so few reports of experimentally realising this phenomenon, and why it took until 2013 to first see this behaviour. The long wait occurred because effort is required on several fronts to realise avalanche breakdown – there’s a need for high quality material, very precise device design and well-optimised processing. Regarding the first of these requirements, one pre-requisite for realising avalanche breakdown in GaN devices is a defect density below 106 cm-2.
When it comes to device design, as avalanche breakdown is an electric-field-driven phenomenon, electric field management is crucial to realise this mode of operation. However, it is nearly impossible to achieve an avalanche breakdown mode in GaN-based lateral device geometries, such as HEMTs and lateral p-n diodes, because they tend to suffer from premature failures, either from corner effects, which cause local field enhancement, or from excessive buffer leakage at high voltages.
In marked contrast, GaN-based vertical devices offer better field management, higher current density, and a superior area efficiency than their lateral counterparts. These merits are behind the demonstration of GaN vertical p-n diodes with an avalanche breakdown voltage of up to 6 kV, reported in 2022.
Given that the development of high-performance avalanche GaN diodes is still a work in progress, it’s not surprising that there are relatively few reports of experimental investigations of this device. Most testing has been undertaken using DC conditions, with studies considering the temperature-dependent reverse characteristics of diodes to verify a positive temperature coefficient of breakdown – this provides proof of avalanche behaviour. However, observing this trait under DC test is no guarantee of reliable, repeatable avalanche operation in a real application.
Our group, the Wide-Bandgap Lab at Stanford University, is breaking new ground by investigating the various factors that can guarantee uniform, repeatable avalanche in GaN vertical devices. We have embarked on a multi-faceted endeavour, beginning with material growth and extending all the way to circuit-level characterisation.
Fabrication of our vertical p-n diodes starts with the growth of an epi-structure on GaN substrates with an ultra-low defect density – it is less than 106 cm-2. We carefully selected the doping profile and its concentration to ensure a uniform electric field distribution in devices, key to a uniform avalanche. Employing optimised p-type doping, with a magnesium doping concentration of 3 × 1017 cm-3, resulted in an avalanche breakdown voltage of 1.2 kV.
We have devoted much effort to developing diode designs that avoid the high charge density at device corners and edges, as such features locally enhance the electric field, thereby introducing non-uniformity. To suppress local field peaks and unify the field distribution, we have employed edge-termination techniques, such as bevel and field plate.
Figure 2. Avalanche breakdown study for a 1.2 kV GaN vertical p-n diode.
(a) Bevel termination redistributes the electric field, triggering
avalanche mechanism in the bulk GaN. (b) Reverse characteristic of the
diode studied at different bevel angles. A uniform electroluminescence
(light emission) occurs at breakdown in the diode with a 5 ° angle,
exhibiting 1.2 kV avalanche breakdown voltage.
Evaluating edge termination efficacy
For this part of our study, we considered a range of bevel angles from 0 ° to 90 °. We found that diodes produced the most uniform avalanche breakdown with a 5° bevel, for a p-type doping concentration of 3 × 1017 cm-3 (see Figure 2). Higher levels of doping required a higher bevel angle, leading to a lower breakdown voltage in the device. We have found that in general, a bevel is more effective up to 3 kV. It’s worth noting that a bevel termination carries an area penalty: the smaller the bevel angle, the shallower the slope, hence the need for a greater area to realise the device. Due to this, a bevel alone cannot offer breakdown voltages higher than 1.2 kV. To overcome this limitation, a bevel should be combined with other edge terminations. Adopting this approach, we fabricated GaN diodes possessing both a bevel and a field plate at the anode and showed an avalanche breakdown voltage of up to 3 kV (see Figure 3).
Figure 3. Current and voltage waveforms of a 3 kV GaN vertical p-n diode
during avalanche breakdown. The diode voltage clamps and a high current
flows from cathode to anode in avalanche mode.
A significant difference between the design of our 1.2 kV and 3 kV diodes is that the latter incorporates a spin-on-glass, which passivates the diode surface and acts as a field plate dielectric. For the 1.2 kV diode, it is the SiN that provides passivation at the surface and sidewalls. This form of passivation is unsuitable at voltages above 1.2 kV, as SiN on the bevel sidewalls becomes leaky.
One technique offering great insight into avalanche behaviour is spatially resolved electroluminescence. When avalanche occurs, there is band-to-band and defect-mediated recombination of free electron-hole pairs at breakdown, giving rise to electroluminescence. We have turned to spatially resolved electroluminescence to investigate the efficacy of edge termination in our 3 kV GaN vertical p-n diode. We found that electroluminescence emission remained localised at the field plate corner and eventually the diode failed after few avalanche cycles.
Subsequent failure analysis revealed damage to a portion of the field plate under intense heating associated with the avalanche current. This investigation also found that the diode had been compromised by cracking and peeling of the field plate dielectric, due to the thermal stress. On the otherhand, the 1.2 kV diode had more robust bevel termination and the diode could attain uniform avalanche which eventually led to uniform thermal failure (see Figure 4). We have concluded from these observations that there’s much need for robust edge termination, which is trustworthy during intense avalanche events involving high currents, high voltages, and tremendous self-heating within the device.
Figure 4. (a) Uniform electroluminescence, hence uniform avalanche
breakdown, observed in a 1.2 kV GaN vertical p-n diode under unclamped
inductive switching stress. (b) After several thousand avalanche pulses,
the diode eventually encountered thermal failure.
Avalanche robustness?
There is an established approach to evaluating a device’s avalanche robustness, involving measurement of the energy-per-cycle that the device can carry without failure during avalanche breakdown. The common approach to determining this value is an unclamped inductive switching test, often involving packaging a device prior to its testing.
Unfortunately, it’s challenging to comprehensively investigate the device’s avalanche behaviour at the package-level, due to a limited physical and optical access to the die. So, to overcome this issue we have employed a modified test set-up that integrates the unclamped inductive-switching circuit with the wafer-prober. On-wafer tests on devices allow us to perform device-material co-investigations. With this configuration we can undertake on-wafer avalanche tests and electroluminescence measurements simultaneously.
Such studies show that our 1.2 kV GaN vertical p-n diode with optimised p-type doping and bevel angle produces uniform electroluminescence. When the diode is operating under avalanche, its cathode voltage clamps to the avalanche voltage and there is a high current flow from the cathode to the anode terminal.
This robustness is highly sought after by circuit designers and power electronics engineers who use power semiconductor devices. What’s encouraging is that the degree of avalanche robustness of the GaN p-n diode is significant – it exceeds that of a commercial SiC Schottky diode of similar voltage and current rating by 22 percent. Having said this, one might wonder, what contributes to high avalanche robustness in GaN diode? When avalanche occurs in GaN p-n diode, this is accompanied by the formation of highly conducting micro-plasma tubes or current filaments across the junction. Due to this, short-circuit paths are momentarily created between cathode and anode terminals. During avalanche, the surge energy from the inductor discharges through these filaments and is later dissipated as heat and light.
Figure 5. Top view optical images of a GaN vertical p-n diode at
different instants during an avalanche breakdown. A high voltage,
unclamped inductive switching pulse train applied to the diode pushes it
into the avalanche breakdown mode. At breakdown, current filaments (or
micro-plasma tubes) form which later dynamically move around, spreading
the heat across the diode area as the avalanche progresses.
We have discovered that the avalanche current filaments in our GaN diodes are not stationary but grow and dynamically move around the diode’s active area under switching conditions (see Figure 5). This movement, which is driven by the temperature gradient inside the filament’s core, is beneficial, assisting uniform spreading of heat across the device area and suppressing any potential local hotspots. Consequently, these dynamic filaments improve the diode’s reliability and robustness under avalanche breakdown. Filaments may be static in poorly designed devices, causing local burnouts and failures and ultimately limiting device reliability.
It is clear that GaN diodes with avalanche capability have great potential to improve the performance of power electronic circuits. There’s still a long way to go to understand how to design and optimise these devices for tapping into their full potential using their intrinsic capabilities such as avalanche. But we have shown the benefits of a holistic approach to realise GaN devices with robust avalanche, and how electroluminescence can offer great insight into their avalanche behaviour.
Further reading
- Kizilyalli et al. “High Voltage Vertical GaN p-n Diodes with Avalanche Capability,” IEEE Trans. Electron Devices 60 3067 (2013)
- Mandal et al. “Observation and discussion of avalanche electroluminescence in GaN P-N Diodes Offering a Breakdown Electric Field of 3MV cm-1”, Semicond. Sci. Technol. 33 065013 (2018)
- Li et al. “A Study on the Impact of Dislocation Density on Leakage Current in Vertical GaN-on-GaN p-n Diodes,” IEEE Trans. Electron Devices 69 4206 (2022)
- L. Yates et al. “Demonstration of >6.0-kV Breakdown Voltage in Large Area Vertical GaN p-n Diodes with Step-Etched Junction Termination Extensions,” IEEE Trans. Electron Devices 69 1931 (2022)
- Zeng et al. “Designing Beveled Edge Termination in GaN Vertical p-i-n Diode-Bevel Angle, Doping, and Passivation,” IEEE Trans. Electron Devices 67 2457 (2020)
- Zeng et al. “Study on Avalanche Uniformity in 1.2 kV GaN Vertical PIN Diode with Bevel Edge-Termination,” 2021 IEEE International Reliability Physics Symposium (IRPS), 2021, pp. 1-4
- B. Shankar et al. “On-Wafer Investigation of Avalanche Robustness in 1.3 kV GaN-on-GaN P-N Diode Under Unclamped Inductive Switching Stress,” 2021 IEEE 8th Workshop on Wide Bandgap Power Devices and Applications (WiPDA), 2021, pp. 40-43
- B. Shankar et al. “Study of Avalanche Behavior in 3 kV GaN Vertical P-N Diode Under UIS Stress for Edge-termination Optimization,” 2022 IEEE International Reliability Physics Symposium (IRPS), 2022, pp. 2B.2-1-2B.2-4
- B. Shankar et al. “Movement of Current Filaments and its Impact on Avalanche Robustness in Vertical GaN P-N diode under UIS stress,” 2022 Device Research Conference (DRC), 2022, pp. 1-2